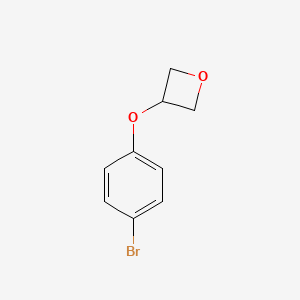
3-(4-Bromophenoxy)oxetane
概要
説明
3-(4-Bromophenoxy)oxetane is an organic compound characterized by the presence of an oxetane ring substituted with a 4-bromophenoxy group. The oxetane ring is a four-membered cyclic ether, which is known for its ring strain and unique reactivity. The incorporation of a bromophenoxy group adds further chemical versatility, making this compound of interest in various fields of research and industry.
作用機序
Target of Action
Oxetanes, in general, have been employed to improve drugs’ physiochemical properties . They are more metabolically stable and lipophilicity neutral . Oxetane is an electron-withdrawing group, reducing the basicity of its adjacent nitrogen atom, which may lower the drug’s overall lipophilicity .
Mode of Action
Oxetanes have been known to disrupt protein microtubule functions in the cell, which pull apart the chromosomes before cell division (mitosis) .
Biochemical Pathways
Oxetanes are known to influence the stability of metabolic pathways and can affect the basicity of adjacent nitrogen atoms .
Pharmacokinetics
Oxetanes are known to be more metabolically stable and have neutral lipophilicity, which could impact their bioavailability .
Action Environment
The formation of the oxetane ring from an epoxide requires moderate heating .
準備方法
Synthetic Routes and Reaction Conditions
The synthesis of 3-(4-Bromophenoxy)oxetane typically involves the reaction of 4-bromophenol with oxetane derivatives. One common method is the nucleophilic substitution reaction where 4-bromophenol reacts with an oxetane precursor in the presence of a base such as sodium hydride or potassium carbonate. The reaction is usually carried out in an aprotic solvent like dimethylformamide or tetrahydrofuran at elevated temperatures to facilitate the formation of the desired product.
Industrial Production Methods
Industrial production of this compound may involve similar synthetic routes but on a larger scale. The process optimization focuses on maximizing yield and purity while minimizing costs and environmental impact. Continuous flow reactors and automated synthesis platforms are often employed to achieve efficient and scalable production.
化学反応の分析
Types of Reactions
3-(4-Bromophenoxy)oxetane undergoes various chemical reactions, including:
Nucleophilic Substitution: The bromine atom can be replaced by other nucleophiles, leading to the formation of diverse derivatives.
Oxidation: The oxetane ring can be oxidized to form more reactive intermediates.
Reduction: The bromophenoxy group can be reduced to form phenoxy derivatives.
Ring-Opening Reactions: The strained oxetane ring is prone to ring-opening reactions, which can be catalyzed by acids or bases.
Common Reagents and Conditions
Nucleophilic Substitution: Sodium hydride, potassium carbonate, dimethylformamide, tetrahydrofuran.
Oxidation: Peroxy acids, such as peroxytrifluoroacetic acid.
Reduction: Hydrogen gas with palladium on carbon catalyst.
Ring-Opening: Acidic or basic catalysts, such as hydrochloric acid or sodium hydroxide.
Major Products Formed
Nucleophilic Substitution: Various substituted oxetane derivatives.
Oxidation: Oxetane oxides.
Reduction: Phenoxy derivatives.
Ring-Opening: Linear or branched ethers.
科学的研究の応用
3-(4-Bromophenoxy)oxetane has several applications in scientific research:
Medicinal Chemistry: Used as a building block for the synthesis of pharmaceutical compounds due to its unique reactivity and structural features.
Material Science: Employed in the development of novel polymers and materials with specific properties.
Chemical Biology: Utilized in the study of biological pathways and mechanisms due to its ability to interact with various biomolecules.
Industrial Chemistry: Applied in the synthesis of specialty chemicals and intermediates for various industrial processes.
類似化合物との比較
Similar Compounds
3-Phenoxyoxetane: Lacks the bromine atom, resulting in different reactivity and biological activity.
3-(4-Chlorophenoxy)oxetane: Contains a chlorine atom instead of bromine, leading to variations in chemical properties and applications.
3-(4-Fluorophenoxy)oxetane:
Uniqueness
3-(4-Bromophenoxy)oxetane is unique due to the presence of the bromine atom, which enhances its reactivity and allows for specific interactions in chemical and biological systems. This makes it a valuable compound for the synthesis of complex molecules and the study of various chemical and biological processes .
特性
IUPAC Name |
3-(4-bromophenoxy)oxetane | |
---|---|---|
Source | PubChem | |
URL | https://pubchem.ncbi.nlm.nih.gov | |
Description | Data deposited in or computed by PubChem | |
InChI |
InChI=1S/C9H9BrO2/c10-7-1-3-8(4-2-7)12-9-5-11-6-9/h1-4,9H,5-6H2 | |
Source | PubChem | |
URL | https://pubchem.ncbi.nlm.nih.gov | |
Description | Data deposited in or computed by PubChem | |
InChI Key |
KTHYKLLJTJDHER-UHFFFAOYSA-N | |
Source | PubChem | |
URL | https://pubchem.ncbi.nlm.nih.gov | |
Description | Data deposited in or computed by PubChem | |
Canonical SMILES |
C1C(CO1)OC2=CC=C(C=C2)Br | |
Source | PubChem | |
URL | https://pubchem.ncbi.nlm.nih.gov | |
Description | Data deposited in or computed by PubChem | |
Molecular Formula |
C9H9BrO2 | |
Source | PubChem | |
URL | https://pubchem.ncbi.nlm.nih.gov | |
Description | Data deposited in or computed by PubChem | |
Molecular Weight |
229.07 g/mol | |
Source | PubChem | |
URL | https://pubchem.ncbi.nlm.nih.gov | |
Description | Data deposited in or computed by PubChem | |
Synthesis routes and methods I
Procedure details
Synthesis routes and methods II
Procedure details
Retrosynthesis Analysis
AI-Powered Synthesis Planning: Our tool employs the Template_relevance Pistachio, Template_relevance Bkms_metabolic, Template_relevance Pistachio_ringbreaker, Template_relevance Reaxys, Template_relevance Reaxys_biocatalysis model, leveraging a vast database of chemical reactions to predict feasible synthetic routes.
One-Step Synthesis Focus: Specifically designed for one-step synthesis, it provides concise and direct routes for your target compounds, streamlining the synthesis process.
Accurate Predictions: Utilizing the extensive PISTACHIO, BKMS_METABOLIC, PISTACHIO_RINGBREAKER, REAXYS, REAXYS_BIOCATALYSIS database, our tool offers high-accuracy predictions, reflecting the latest in chemical research and data.
Strategy Settings
Precursor scoring | Relevance Heuristic |
---|---|
Min. plausibility | 0.01 |
Model | Template_relevance |
Template Set | Pistachio/Bkms_metabolic/Pistachio_ringbreaker/Reaxys/Reaxys_biocatalysis |
Top-N result to add to graph | 6 |
Feasible Synthetic Routes
試験管内研究製品の免責事項と情報
BenchChemで提示されるすべての記事および製品情報は、情報提供を目的としています。BenchChemで購入可能な製品は、生体外研究のために特別に設計されています。生体外研究は、ラテン語の "in glass" に由来し、生物体の外で行われる実験を指します。これらの製品は医薬品または薬として分類されておらず、FDAから任何の医療状態、病気、または疾患の予防、治療、または治癒のために承認されていません。これらの製品を人間または動物に体内に導入する形態は、法律により厳格に禁止されています。これらのガイドラインに従うことは、研究と実験において法的および倫理的な基準の遵守を確実にするために重要です。