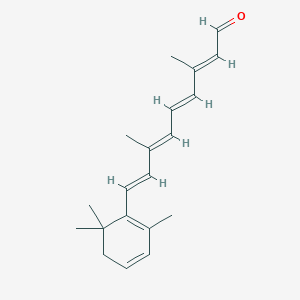
Dehydroretinaldehyde
概要
説明
Dehydroretinaldehyde, also known as 3,4-dehydroretinal, is a derivative metabolite of retinal. It belongs to the group of vitamin A2 compounds and is a retinaldehyde form. This compound is endogenously present in some species, including certain freshwater fishes and some fish found in India . It plays a crucial role in the visual processes of these species, acting as a chromophore in visual pigments.
準備方法
Synthetic Routes and Reaction Conditions: Dehydroretinaldehyde can be synthesized through various chemical reactions. One common method involves the saponification of 5,6-monoepoxy-3-dehydroretinyl acetate with ethanolic potassium hydroxide (KOH) to yield the epoxy alcohol. This is then oxidized with manganese dioxide (MnO2) to produce 3-dehydroretinal .
Industrial Production Methods: While specific industrial production methods for 3-dehydroretinal are not widely documented, the synthesis typically involves large-scale chemical reactions similar to those used in laboratory settings. The process would likely include the use of high-pressure liquid chromatography (HPLC) for purification and quality control .
化学反応の分析
Types of Reactions: Dehydroretinaldehyde undergoes various chemical reactions, including:
Oxidation: Conversion to 3-dehydroretinoic acid.
Reduction: Formation of 3-dehydroretinol.
Isomerization: Conversion between different isomeric forms, such as 11-cis and all-trans forms.
Common Reagents and Conditions:
Oxidation: Manganese dioxide (MnO2) is commonly used.
Reduction: Dehydrogenases are employed for the reduction process.
Isomerization: Enzymatic catalysis is often involved.
Major Products:
- 3-Dehydroretinoic acid
- 3-Dehydroretinol
- Various isomeric forms of 3-dehydroretinal
科学的研究の応用
Biochemical Pathways and Retinoid Metabolism
Dehydroretinaldehyde plays a critical role in the metabolism of retinoids, which are vital for various biological processes, including vision and cellular differentiation. It is involved in the conversion of retinol to retinaldehyde and subsequently to retinoic acid (RA). The enzyme retinaldehyde dehydrogenase (RALDH) is crucial in this pathway, as it catalyzes the oxidation of retinaldehyde to RA, influencing gene expression during development.
Table 1: Key Enzymes in Retinoid Metabolism
Enzyme | Substrate | Product | Role in Metabolism |
---|---|---|---|
Alcohol Dehydrogenase (ADH1) | Retinol | Retinaldehyde | First step in retinol clearance |
Retinaldehyde Dehydrogenase (RALDH) | Retinaldehyde | Retinoic Acid | Second step in retinol clearance |
P450 27C1 | All-trans Retinoids | Dehydroretinoids | Desaturation of retinoids |
Therapeutic Applications in Cancer Treatment
Recent studies have highlighted the potential of this compound as an anticancer agent. Research indicates that inhibiting aldehyde dehydrogenase (ALDH), which typically detoxifies retinaldehyde, can enhance the cytotoxic effects of retinaldehyde on cancer cells. This suggests that this compound could be leveraged to improve therapeutic outcomes in cancer treatment.
Case Study: ALDH Inhibition and Retinaldehyde Cytotoxicity
In a study involving A549 lung cancer cells, the combination of retinaldehyde with the ALDH inhibitor DEAB significantly increased reactive oxygen species (ROS) production and reduced cell viability. The results demonstrated that targeting ALDH could potentiate the effects of retinaldehyde, making it a promising candidate for further research in cancer therapies .
Developmental Biology and Teratogenic Effects
This compound's role extends into developmental biology, where it is implicated in the regulation of embryonic development through its conversion to retinoic acid. Disruptions in this pathway can lead to teratogenic effects, as evidenced by studies showing that RALDH inhibition can result in abnormal eye development in model organisms .
Table 2: Impact of RALDH Inhibition on Development
Study | Model Organism | Observed Effects |
---|---|---|
Raldh1 Knockout Mice | Mice | Reduced RA synthesis; eye defects |
RALDH Inhibition | Zebrafish | Abnormal craniofacial development |
Potential Applications in Stem Cell Research
This compound may also find applications in stem cell research. Retinoic acid is known to influence stem cell differentiation; thus, understanding how this compound affects this process could lead to advancements in regenerative medicine and therapeutic strategies for conditions like leukemia .
作用機序
Dehydroretinaldehyde functions as a chromophore in visual pigments. Upon absorption of light, it undergoes photoisomerization, changing from the 11-cis form to the all-trans form. This change in shape triggers a conformational change in the associated opsin protein, leading to a signal transduction pathway that ultimately results in visual perception . The molecular targets include opsin proteins, and the pathways involved are part of the visual cycle .
類似化合物との比較
- Retinal (Vitamin A1)
- 3,4-Dehydroretinol
- 3,4-Dehydroretinoic acid
Comparison: Dehydroretinaldehyde is unique in its specific role in the visual systems of certain species. While retinal (Vitamin A1) is more commonly found in mammals, 3-dehydroretinal is prevalent in certain fish species and plays a similar role in their visual processes . The presence of an additional double bond in 3-dehydroretinal distinguishes it from retinal, affecting its absorption properties and reactivity .
生物活性
Dehydroretinaldehyde, a derivative of retinal, plays a crucial role in various biological processes, particularly in the metabolism of retinoids. This article explores the biological activity of this compound, focusing on its enzymatic interactions, physiological roles, and implications in health and disease.
Overview of this compound
This compound, specifically all-trans 3,4-dehydroretinal (vitamin A2), is formed through the action of cytochrome P450 enzymes, particularly P450 27C1. This compound is significant in the retinoid metabolic pathway, contributing to the pool of active retinoids in various tissues, including skin and retina. The conversion of retinol to this compound involves desaturation reactions that are catalyzed by specific enzymes.
Cytochrome P450 27C1
P450 27C1 is identified as a key enzyme responsible for the desaturation of retinol to this compound. Research indicates that this enzyme exhibits high catalytic efficiency for retinoid substrates:
- Catalytic Efficiency : The efficiency of P450 27C1 for all-trans retinol desaturation is approximately , significantly higher than related enzymes like rabbit P450 2B4 .
- Substrate Specificity : P450 27C1 can also desaturate other retinoids such as retinal and retinoic acid, indicating its versatile role in retinoid metabolism .
Aldehyde Dehydrogenases (ALDHs)
Aldehyde dehydrogenases are another class of enzymes that play a crucial role in converting retinaldehyde to retinoic acid (RA), a biologically active form of vitamin A. The activity of these enzymes is essential for maintaining proper levels of retinoic acid in tissues:
- ALDH Activity : Studies show that specific ALDH isoforms are involved in the metabolism of retinaldehyde, with ALDH1A1 being particularly significant in regulating RA synthesis .
- Impact on Cancer Cells : Recent developments have introduced probes like LEI-945 that help profile ALDH activity in cancer cells, showcasing the importance of retinaldehyde metabolism in oncological contexts .
Retinoid Signaling
This compound and its derivatives influence several physiological processes through retinoid signaling pathways:
- Vision : In vertebrates, this compound contributes to phototransduction processes by participating in the formation of visual pigments .
- Development : Retinoids are crucial morphogens during embryonic development, regulating gene expression patterns necessary for proper organogenesis .
Metabolic Regulation
Research indicates that retinoid metabolism impacts metabolic pathways:
- Gluconeogenesis : Aldh1a1 has been implicated in coordinating hepatic gluconeogenesis by regulating RA levels, which affects glucose homeostasis .
- Toxicity Management : Genetic studies reveal that enzymes involved in retinaldehyde metabolism protect against vitamin A toxicity by facilitating its clearance from tissues .
Case Studies and Research Findings
Several studies highlight the biological significance of this compound:
特性
IUPAC Name |
(2E,4E,6E,8E)-3,7-dimethyl-9-(2,6,6-trimethylcyclohexa-1,3-dien-1-yl)nona-2,4,6,8-tetraenal | |
---|---|---|
Source | PubChem | |
URL | https://pubchem.ncbi.nlm.nih.gov | |
Description | Data deposited in or computed by PubChem | |
InChI |
InChI=1S/C20H26O/c1-16(8-6-9-17(2)13-15-21)11-12-19-18(3)10-7-14-20(19,4)5/h6-13,15H,14H2,1-5H3/b9-6+,12-11+,16-8+,17-13+ | |
Source | PubChem | |
URL | https://pubchem.ncbi.nlm.nih.gov | |
Description | Data deposited in or computed by PubChem | |
InChI Key |
QHNVWXUULMZJKD-OVSJKPMPSA-N | |
Source | PubChem | |
URL | https://pubchem.ncbi.nlm.nih.gov | |
Description | Data deposited in or computed by PubChem | |
Canonical SMILES |
CC1=C(C(CC=C1)(C)C)C=CC(=CC=CC(=CC=O)C)C | |
Source | PubChem | |
URL | https://pubchem.ncbi.nlm.nih.gov | |
Description | Data deposited in or computed by PubChem | |
Isomeric SMILES |
CC1=C(C(CC=C1)(C)C)/C=C/C(=C/C=C/C(=C/C=O)/C)/C | |
Source | PubChem | |
URL | https://pubchem.ncbi.nlm.nih.gov | |
Description | Data deposited in or computed by PubChem | |
Molecular Formula |
C20H26O | |
Source | PubChem | |
URL | https://pubchem.ncbi.nlm.nih.gov | |
Description | Data deposited in or computed by PubChem | |
DSSTOX Substance ID |
DTXSID30878517 | |
Record name | 3-Dehydroretinal | |
Source | EPA DSSTox | |
URL | https://comptox.epa.gov/dashboard/DTXSID30878517 | |
Description | DSSTox provides a high quality public chemistry resource for supporting improved predictive toxicology. | |
Molecular Weight |
282.4 g/mol | |
Source | PubChem | |
URL | https://pubchem.ncbi.nlm.nih.gov | |
Description | Data deposited in or computed by PubChem | |
Physical Description |
Solid | |
Record name | Vitamin A2 aldehyde | |
Source | Human Metabolome Database (HMDB) | |
URL | http://www.hmdb.ca/metabolites/HMDB0035695 | |
Description | The Human Metabolome Database (HMDB) is a freely available electronic database containing detailed information about small molecule metabolites found in the human body. | |
Explanation | HMDB is offered to the public as a freely available resource. Use and re-distribution of the data, in whole or in part, for commercial purposes requires explicit permission of the authors and explicit acknowledgment of the source material (HMDB) and the original publication (see the HMDB citing page). We ask that users who download significant portions of the database cite the HMDB paper in any resulting publications. | |
CAS No. |
472-87-7 | |
Record name | Dehydroretinal | |
Source | CAS Common Chemistry | |
URL | https://commonchemistry.cas.org/detail?cas_rn=472-87-7 | |
Description | CAS Common Chemistry is an open community resource for accessing chemical information. Nearly 500,000 chemical substances from CAS REGISTRY cover areas of community interest, including common and frequently regulated chemicals, and those relevant to high school and undergraduate chemistry classes. This chemical information, curated by our expert scientists, is provided in alignment with our mission as a division of the American Chemical Society. | |
Explanation | The data from CAS Common Chemistry is provided under a CC-BY-NC 4.0 license, unless otherwise stated. | |
Record name | 3-Dehydroretinal | |
Source | ChemIDplus | |
URL | https://pubchem.ncbi.nlm.nih.gov/substance/?source=chemidplus&sourceid=0000472877 | |
Description | ChemIDplus is a free, web search system that provides access to the structure and nomenclature authority files used for the identification of chemical substances cited in National Library of Medicine (NLM) databases, including the TOXNET system. | |
Record name | 3-Dehydroretinal | |
Source | EPA DSSTox | |
URL | https://comptox.epa.gov/dashboard/DTXSID30878517 | |
Description | DSSTox provides a high quality public chemistry resource for supporting improved predictive toxicology. | |
Record name | Dehydroretinaldehyde | |
Source | European Chemicals Agency (ECHA) | |
URL | https://echa.europa.eu/substance-information/-/substanceinfo/100.006.781 | |
Description | The European Chemicals Agency (ECHA) is an agency of the European Union which is the driving force among regulatory authorities in implementing the EU's groundbreaking chemicals legislation for the benefit of human health and the environment as well as for innovation and competitiveness. | |
Explanation | Use of the information, documents and data from the ECHA website is subject to the terms and conditions of this Legal Notice, and subject to other binding limitations provided for under applicable law, the information, documents and data made available on the ECHA website may be reproduced, distributed and/or used, totally or in part, for non-commercial purposes provided that ECHA is acknowledged as the source: "Source: European Chemicals Agency, http://echa.europa.eu/". Such acknowledgement must be included in each copy of the material. ECHA permits and encourages organisations and individuals to create links to the ECHA website under the following cumulative conditions: Links can only be made to webpages that provide a link to the Legal Notice page. | |
Record name | 3-DEHYDRORETINAL | |
Source | FDA Global Substance Registration System (GSRS) | |
URL | https://gsrs.ncats.nih.gov/ginas/app/beta/substances/HV8T003XO1 | |
Description | The FDA Global Substance Registration System (GSRS) enables the efficient and accurate exchange of information on what substances are in regulated products. Instead of relying on names, which vary across regulatory domains, countries, and regions, the GSRS knowledge base makes it possible for substances to be defined by standardized, scientific descriptions. | |
Explanation | Unless otherwise noted, the contents of the FDA website (www.fda.gov), both text and graphics, are not copyrighted. They are in the public domain and may be republished, reprinted and otherwise used freely by anyone without the need to obtain permission from FDA. Credit to the U.S. Food and Drug Administration as the source is appreciated but not required. | |
Record name | Vitamin A2 aldehyde | |
Source | Human Metabolome Database (HMDB) | |
URL | http://www.hmdb.ca/metabolites/HMDB0035695 | |
Description | The Human Metabolome Database (HMDB) is a freely available electronic database containing detailed information about small molecule metabolites found in the human body. | |
Explanation | HMDB is offered to the public as a freely available resource. Use and re-distribution of the data, in whole or in part, for commercial purposes requires explicit permission of the authors and explicit acknowledgment of the source material (HMDB) and the original publication (see the HMDB citing page). We ask that users who download significant portions of the database cite the HMDB paper in any resulting publications. | |
Melting Point |
77 - 78 °C | |
Record name | Vitamin A2 aldehyde | |
Source | Human Metabolome Database (HMDB) | |
URL | http://www.hmdb.ca/metabolites/HMDB0035695 | |
Description | The Human Metabolome Database (HMDB) is a freely available electronic database containing detailed information about small molecule metabolites found in the human body. | |
Explanation | HMDB is offered to the public as a freely available resource. Use and re-distribution of the data, in whole or in part, for commercial purposes requires explicit permission of the authors and explicit acknowledgment of the source material (HMDB) and the original publication (see the HMDB citing page). We ask that users who download significant portions of the database cite the HMDB paper in any resulting publications. | |
Retrosynthesis Analysis
AI-Powered Synthesis Planning: Our tool employs the Template_relevance Pistachio, Template_relevance Bkms_metabolic, Template_relevance Pistachio_ringbreaker, Template_relevance Reaxys, Template_relevance Reaxys_biocatalysis model, leveraging a vast database of chemical reactions to predict feasible synthetic routes.
One-Step Synthesis Focus: Specifically designed for one-step synthesis, it provides concise and direct routes for your target compounds, streamlining the synthesis process.
Accurate Predictions: Utilizing the extensive PISTACHIO, BKMS_METABOLIC, PISTACHIO_RINGBREAKER, REAXYS, REAXYS_BIOCATALYSIS database, our tool offers high-accuracy predictions, reflecting the latest in chemical research and data.
Strategy Settings
Precursor scoring | Relevance Heuristic |
---|---|
Min. plausibility | 0.01 |
Model | Template_relevance |
Template Set | Pistachio/Bkms_metabolic/Pistachio_ringbreaker/Reaxys/Reaxys_biocatalysis |
Top-N result to add to graph | 6 |
Feasible Synthetic Routes
Q1: What is the molecular formula and weight of 3-dehydroretinal?
A1: 3-Dehydroretinal, also known as vitamin A2 aldehyde, has a molecular formula of C20H26O and a molecular weight of 282.43 g/mol.
Q2: How does the structure of 3-dehydroretinal differ from retinal (retinaldehyde)?
A2: 3-Dehydroretinal possesses an additional double bond in the ring structure compared to retinal, specifically between carbons 3 and 4. This structural difference leads to a shift in its absorption spectrum towards longer wavelengths. []
Q3: What are the spectroscopic properties of 3-dehydroretinal?
A3: 3-Dehydroretinal exhibits characteristic absorption bands in the ultraviolet-visible (UV-Vis) region. Its absorption maximum (λmax) typically lies between 350 nm and 500 nm, depending on factors such as the solvent and its interaction with proteins like opsin. [, , ]
Q4: How does the absorption spectrum of 3-dehydroretinal compare to that of retinal?
A4: The presence of the additional double bond in 3-dehydroretinal causes its absorption spectrum to be red-shifted compared to retinal. This means it absorbs light at longer wavelengths, enabling organisms to see further into the red end of the spectrum. [, , ]
Q5: What is the role of 3-dehydroretinal in vision?
A5: 3-Dehydroretinal, like retinal, acts as a chromophore in visual pigments. It forms a Schiff base linkage with a lysine residue in the opsin protein, forming a light-sensitive molecule. [, , ]
Q6: What is porphyropsin, and how is it related to 3-dehydroretinal?
A6: Porphyropsin is a visual pigment found predominantly in freshwater fish and some crustaceans. It is formed when 3-dehydroretinal binds to opsin. [, , ]
Q7: How does the spectral sensitivity of porphyropsin differ from rhodopsin?
A7: Porphyropsin, containing 3-dehydroretinal, has an absorption maximum at a longer wavelength compared to rhodopsin, which contains retinal. This difference allows organisms with porphyropsin to have greater sensitivity to light in the yellow-green region of the spectrum. [, , ]
Q8: Do all crayfish have porphyropsin in their eyes?
A8: Not necessarily. Studies have shown that the ratio of rhodopsin to porphyropsin can vary significantly between individual crayfish and even within different ommatidia of the same eye. This suggests a complex regulation of retinoid metabolism at the individual ommatidium level. []
Q9: Can 3-dehydroretinal replace retinal in other visual pigments?
A9: Yes, research has shown that 3-dehydroretinal can substitute retinal in some visual pigments. For example, in squid retinochrome, both retinal and 3-dehydroretinal can act as chromophores, indicating a degree of flexibility in the chromophore binding site. []
Q10: Does the conformation of 3-dehydroretinal change upon binding to opsin?
A11: Yes, Resonance Raman spectroscopy studies on bacteriorhodopsin analogues suggest that the conformation of the 3-dehydroretinal chromophore, specifically the Schiff base region, differs between the unphotolysed state (BR570) and the 13-cis component (BR548). This indicates that interactions with the protein environment influence chromophore conformation. []
Q11: Can modifications to the 3-dehydroretinal structure alter the spectral sensitivity of visual pigments?
A12: Yes, studies using modified 3-dehydroretinal analogues have demonstrated that structural changes can significantly impact spectral sensitivity. For instance, incorporating a 3-methoxy group and a fluorine atom at position 14 led to the creation of a red-shifted visual pigment analogue with an absorption maximum at 720 nm. []
Q12: How is 3-dehydroretinal produced in organisms?
A13: 3-Dehydroretinal is synthesized from vitamin A2 (3-dehydroretinol), which itself is either obtained from the diet or converted from vitamin A1 (retinol) through enzymatic modifications. [, ]
Q13: What factors influence the ratio of 3-dehydroretinal to retinal in an organism?
A13: Several factors can influence this ratio, including:
- Diet: Availability of dietary precursors like vitamin A1 and A2. []
- Species: Inherent metabolic capabilities of different species. []
- Environment: Adaptations to specific light environments. Freshwater species often have higher 3-dehydroretinal levels, maximizing sensitivity to light prevalent in their habitat. [, ]
- Season: Seasonal variations in light conditions can trigger shifts in the ratio, as observed in crayfish. []
Q14: Can 3-dehydroretinal be metabolized into other compounds?
A14: Yes, similar to retinal, 3-dehydroretinal can be metabolized into various forms, including:
- Reduction to 3-dehydroretinol: This reaction is crucial for the storage and transport of vitamin A2. []
- Oxidation to 3-dehydroretinoic acid: This pathway plays a role in regulating gene expression and cell differentiation. []
Q15: What methods are commonly used to identify and quantify 3-dehydroretinal?
A15: Various analytical techniques are employed, including:
- High-performance liquid chromatography (HPLC): This versatile method separates and quantifies different retinoids, including 3-dehydroretinal isomers, with high sensitivity and resolution. [, , , , , ]
- UV-Vis spectrophotometry: This technique measures the absorbance of light at specific wavelengths, providing information about the chromophore composition and concentration in samples. [, , ]
- Resonance Raman spectroscopy: This technique selectively probes the vibrational modes of the chromophore, offering valuable insights into its conformation and interactions with the protein environment. [, , ]
Q16: What are some potential applications of 3-dehydroretinal research?
A16: Research on 3-dehydroretinal and its derivatives holds promise for various applications, including:
試験管内研究製品の免責事項と情報
BenchChemで提示されるすべての記事および製品情報は、情報提供を目的としています。BenchChemで購入可能な製品は、生体外研究のために特別に設計されています。生体外研究は、ラテン語の "in glass" に由来し、生物体の外で行われる実験を指します。これらの製品は医薬品または薬として分類されておらず、FDAから任何の医療状態、病気、または疾患の予防、治療、または治癒のために承認されていません。これらの製品を人間または動物に体内に導入する形態は、法律により厳格に禁止されています。これらのガイドラインに従うことは、研究と実験において法的および倫理的な基準の遵守を確実にするために重要です。