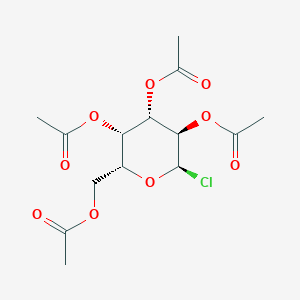
2,3,4,6-Tetra-O-acetyl-α-D-galactopyranosyl chloride
- 専門家チームからの見積もりを受け取るには、QUICK INQUIRYをクリックしてください。
- 品質商品を競争力のある価格で提供し、研究に集中できます。
説明
2,3,4,6-Tetra-o-acetyl-alpha-d-galactopyranosyl chloride is a chemical compound with the molecular formula C14H19ClO9. It is a derivative of galactose, a type of sugar, and is often used in organic synthesis and biochemical research. This compound is known for its role in glycosylation reactions, where it serves as a glycosyl donor.
科学的研究の応用
2,3,4,6-Tetra-o-acetyl-alpha-d-galactopyranosyl chloride has a wide range of applications in scientific research:
Chemistry: Used in the synthesis of complex carbohydrates and glycoconjugates.
Biology: Employed in the study of glycosylation processes and the role of carbohydrates in biological systems.
Medicine: Investigated for its potential in drug development, particularly in the synthesis of glycosylated drugs and prodrugs.
Industry: Utilized in the production of specialty chemicals and materials, including biodegradable polymers and surfactants.
作用機序
Target of Action
2,3,4,6-Tetra-O-acetyl-alpha-D-galactopyranosyl chloride is a chemical compound that is primarily used as an intermediate in the synthesis of various pharmaceuticals and agrochemicals . It is particularly useful in the preparation of glycosides .
Mode of Action
The compound acts as an organic condensation reagent in chemistries such as N- and S- galactosylation reactions . It is involved in the synthesis of spacer-equipped phosphorylated di-, tri-, and tetrasaccharide fragments of the O-specific polysaccharides .
Biochemical Pathways
The compound is involved in the biochemical pathways related to the synthesis of glycosides . Glycosides are molecules in which a sugar is bound to a non-carbohydrate moiety, usually a small organic molecule. Glycosides play numerous important roles in living organisms. Many plants store chemicals in the form of inactive glycosides. These can be activated by enzyme hydrolysis.
Result of Action
The result of the action of 2,3,4,6-Tetra-O-acetyl-alpha-D-galactopyranosyl chloride is the formation of glycosides . These glycosides can be used in the creation of various pharmaceuticals and agrochemicals .
Action Environment
The action, efficacy, and stability of 2,3,4,6-Tetra-O-acetyl-alpha-D-galactopyranosyl chloride can be influenced by various environmental factors. These include temperature, pH, and the presence of other chemicals or compounds . For instance, it is sensitive to temperature and should be stored at -20°C . It is also sensitive to moisture .
生化学分析
Biochemical Properties
It is known to be used in N- and S- galactosylation reactions . This suggests that it may interact with enzymes, proteins, and other biomolecules involved in these reactions.
Cellular Effects
Given its role in galactosylation reactions, it may influence cell function by modifying the structure and function of proteins and other biomolecules .
Molecular Mechanism
It is likely to exert its effects at the molecular level through its role in galactosylation reactions, potentially influencing enzyme activity, protein structure, and gene expression .
Metabolic Pathways
The metabolic pathways involving 2,3,4,6-Tetra-o-acetyl-alpha-d-galactopyranosyl chloride are not well-characterized. It is known to be involved in galactosylation reactions, suggesting it may interact with enzymes and cofactors involved in these pathways .
準備方法
Synthetic Routes and Reaction Conditions
The synthesis of 2,3,4,6-Tetra-o-acetyl-alpha-d-galactopyranosyl chloride typically involves the acetylation of galactose followed by the introduction of a chloride group. One common method includes the following steps:
Acetylation: Galactose is reacted with acetic anhydride in the presence of a catalyst such as sodium acetate to form tetra-acetylated galactose.
Chlorination: The tetra-acetylated galactose is then treated with thionyl chloride (SOCl2) to introduce the chloride group, resulting in the formation of 2,3,4,6-Tetra-o-acetyl-alpha-d-galactopyranosyl chloride.
Industrial Production Methods
Industrial production of this compound follows similar synthetic routes but on a larger scale. The reaction conditions are optimized for higher yields and purity, often involving continuous flow reactors and advanced purification techniques.
化学反応の分析
Types of Reactions
2,3,4,6-Tetra-o-acetyl-alpha-d-galactopyranosyl chloride undergoes several types of chemical reactions, including:
Substitution Reactions: It can participate in nucleophilic substitution reactions where the chloride group is replaced by other nucleophiles.
Hydrolysis: The compound can be hydrolyzed to remove the acetyl groups, yielding galactose derivatives.
Glycosylation: It acts as a glycosyl donor in glycosylation reactions, forming glycosidic bonds with acceptor molecules.
Common Reagents and Conditions
Nucleophilic Substitution: Common nucleophiles include alcohols, amines, and thiols. The reactions are typically carried out in the presence of a base such as pyridine.
Hydrolysis: Acidic or basic conditions can be used to hydrolyze the acetyl groups.
Glycosylation: Catalysts such as silver triflate (AgOTf) or boron trifluoride etherate (BF3·Et2O) are often used to facilitate the glycosylation process.
Major Products
Substitution Reactions: Products depend on the nucleophile used, resulting in various substituted galactose derivatives.
Hydrolysis: The major product is deacetylated galactose.
Glycosylation: The major products are glycosides, where the galactose unit is linked to another molecule via a glycosidic bond.
類似化合物との比較
Similar Compounds
2,3,4,6-Tetra-o-acetyl-alpha-d-galactopyranosyl bromide: Similar in structure but contains a bromide group instead of a chloride group.
2,3,4,6-Tetra-o-acetyl-alpha-d-glucopyranosyl chloride: Similar in structure but derived from glucose instead of galactose.
2,3,4,6-Tetra-o-acetyl-alpha-d-mannopyranosyl chloride: Similar in structure but derived from mannose instead of galactose.
Uniqueness
2,3,4,6-Tetra-o-acetyl-alpha-d-galactopyranosyl chloride is unique due to its specific configuration and reactivity as a glycosyl donor. Its ability to participate in selective glycosylation reactions makes it valuable in the synthesis of complex carbohydrates and glycoconjugates, which are important in various biological and industrial applications.
特性
IUPAC Name |
[(2R,3S,4S,5R,6R)-3,4,5-triacetyloxy-6-chlorooxan-2-yl]methyl acetate |
Source
|
---|---|---|
Source | PubChem | |
URL | https://pubchem.ncbi.nlm.nih.gov | |
Description | Data deposited in or computed by PubChem | |
InChI |
InChI=1S/C14H19ClO9/c1-6(16)20-5-10-11(21-7(2)17)12(22-8(3)18)13(14(15)24-10)23-9(4)19/h10-14H,5H2,1-4H3/t10-,11+,12+,13-,14+/m1/s1 |
Source
|
Source | PubChem | |
URL | https://pubchem.ncbi.nlm.nih.gov | |
Description | Data deposited in or computed by PubChem | |
InChI Key |
BYWPSIUIJNAJDV-HTOAHKCRSA-N |
Source
|
Source | PubChem | |
URL | https://pubchem.ncbi.nlm.nih.gov | |
Description | Data deposited in or computed by PubChem | |
Canonical SMILES |
CC(=O)OCC1C(C(C(C(O1)Cl)OC(=O)C)OC(=O)C)OC(=O)C |
Source
|
Source | PubChem | |
URL | https://pubchem.ncbi.nlm.nih.gov | |
Description | Data deposited in or computed by PubChem | |
Isomeric SMILES |
CC(=O)OC[C@@H]1[C@@H]([C@@H]([C@H]([C@H](O1)Cl)OC(=O)C)OC(=O)C)OC(=O)C |
Source
|
Source | PubChem | |
URL | https://pubchem.ncbi.nlm.nih.gov | |
Description | Data deposited in or computed by PubChem | |
Molecular Formula |
C14H19ClO9 |
Source
|
Source | PubChem | |
URL | https://pubchem.ncbi.nlm.nih.gov | |
Description | Data deposited in or computed by PubChem | |
Molecular Weight |
366.75 g/mol |
Source
|
Source | PubChem | |
URL | https://pubchem.ncbi.nlm.nih.gov | |
Description | Data deposited in or computed by PubChem | |
試験管内研究製品の免責事項と情報
BenchChemで提示されるすべての記事および製品情報は、情報提供を目的としています。BenchChemで購入可能な製品は、生体外研究のために特別に設計されています。生体外研究は、ラテン語の "in glass" に由来し、生物体の外で行われる実験を指します。これらの製品は医薬品または薬として分類されておらず、FDAから任何の医療状態、病気、または疾患の予防、治療、または治癒のために承認されていません。これらの製品を人間または動物に体内に導入する形態は、法律により厳格に禁止されています。これらのガイドラインに従うことは、研究と実験において法的および倫理的な基準の遵守を確実にするために重要です。