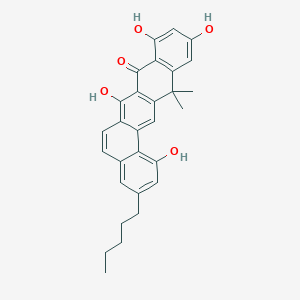
Benastatin C
概要
説明
ベナスタチン C は、放線菌 Streptomyces sp. MI384-DF12 によって生産されるポリケチド合成酵素由来化合物です。 グルタチオン S-トランスフェラーゼの阻害および膵臓リパーゼのエステラーゼ活性の阻害など、さまざまな生物活性で知られています . ベナスタチン C の構造は、NMR 研究により 2-デカルボキシベナスタチン A として解明されました .
準備方法
ベナスタチン C は、Streptomyces sp. MI384-DF12 の培養液から単離されます。 生合成には、テトラデカケチドと 2 つのメチオニン単位が関与しています . ベナスタチン C の調製には、ベナスタチン A の脱炭酸反応が含まれます . 工業的生産方法では、通常、収量を最大化するために最適化された培養条件を用いた発酵プロセスが採用されます .
化学反応の分析
ベナスタチン C は、次のようなさまざまな化学反応を起こします。
酸化: 特定の条件下で酸化して、さまざまな誘導体を生成することができます。
還元: 還元反応は、官能基を修飾することができます。
置換: 置換反応は、分子に新しい官能基を導入することができます。
これらの反応で使用される一般的な試薬には、過マンガン酸カリウムなどの酸化剤、水素化ホウ素ナトリウムなどの還元剤、および置換反応のためのさまざまな触媒が含まれます。 これらの反応で生成される主な生成物は、使用される特定の条件と試薬によって異なります .
科学研究への応用
ベナスタチン C は、いくつかの科学研究への応用があります。
化学: ポリケチド生合成と酵素阻害の研究のためのモデル化合物として使用されます。
生物学: 解毒過程に関与する酵素であるグルタチオン S-トランスフェラーゼを阻害することが示されています.
科学的研究の応用
Benastatin C has several scientific research applications:
Chemistry: It is used as a model compound for studying polyketide biosynthesis and enzyme inhibition.
Industry: It is used in the development of enzyme inhibitors and other bioactive compounds.
作用機序
ベナスタチン C は、主にグルタチオン S-トランスフェラーゼの阻害を通じて効果を発揮します。この酵素は、異物の解毒と酸化ストレスからの保護において重要な役割を果たします。 この酵素を阻害することで、ベナスタチン C は酸化ストレスやその他の環境要因に対する細胞応答を調節することができます . また、マウスリンパ球の芽球化を刺激し、免疫調節効果の可能性を示唆しています .
類似化合物の比較
ベナスタチン C は、ベナスタチン A やベナスタチン B などの化合物を含むベナスタチンファミリーの一部です。 これらの化合物は、類似したポリケチド構造を共有していますが、官能基と生物活性は異なります . 塩素化誘導体であるベナスタチン K も同定されており、独自の生物学的特性を示しています .
類似化合物
ベナスタチン A: 類似した構造ですが、カルボキシル基を持っています。
ベナスタチン B: 異なる官能基を持つ別の誘導体です。
ベナスタチン K: 異なる生物学的活性を持つ塩素化誘導体です.
類似化合物との比較
Benastatin C is part of the benastatin family, which includes compounds like benastatin A and benastatin B. These compounds share a similar polyketide structure but differ in their functional groups and biological activities . Benastatin K, a chlorinated derivative, has also been identified and exhibits unique biological properties .
Similar Compounds
Benastatin A: Similar structure but with a carboxyl group.
Benastatin B: Another derivative with different functional groups.
Benastatin K: A chlorinated derivative with distinct biological activities.
生物活性
Benastatin C is a noteworthy compound derived from the Streptomyces species, specifically identified as a polyketide synthase product. Its biological activities have garnered attention in various fields, particularly due to its interactions with glutathione S-transferases (GSTs) and its potential therapeutic applications. This article provides a comprehensive overview of the biological activity of this compound, supported by research findings, data tables, and case studies.
This compound has been structurally characterized as 2-decarboxy-benastatin A through nuclear magnetic resonance (NMR) studies. This structural identification is crucial for understanding its biological interactions. The compound exhibits significant inhibitory activity against human pi class glutathione S-transferase (GST pi), which plays a vital role in detoxifying harmful compounds in the body .
Mechanism of Action:
- GST Inhibition: this compound inhibits GST pi, leading to altered cellular responses to oxidative stress and apoptosis .
- Stimulation of Lymphocyte Activity: It has been observed to enhance murine lymphocyte blastogenesis, indicating potential immunomodulatory effects .
Biological Activities
The following sections detail the various biological activities associated with this compound, including its cytotoxic effects and influence on cell cycle regulation.
Inhibition of Glutathione S-Transferase (GST)
This compound demonstrates potent inhibitory effects on GSTs, which are critical in the metabolism of xenobiotics and endogenous compounds. This inhibition can lead to increased susceptibility of cancer cells to chemotherapeutic agents.
Induction of Apoptosis
Research indicates that this compound may induce apoptosis in certain cancer cell lines through mechanisms that are not solely dependent on GST inhibition.
- Case Study: In mouse colon 26 adenocarcinoma cells, treatment with this compound resulted in significant cell death over time, suggesting an apoptotic mechanism independent of direct GST inhibition .
- Cell Cycle Arrest: Flow cytometric analysis revealed that this compound can block the cell cycle at the G1/G0 phase, contributing to its antitumor properties .
Case Studies
Several case studies have highlighted the therapeutic potential of this compound in cancer treatment:
- Mouse Colon 26 Cells:
- Combination Therapies:
特性
IUPAC Name |
1,7,9,11-tetrahydroxy-13,13-dimethyl-3-pentylbenzo[a]tetracen-8-one | |
---|---|---|
Source | PubChem | |
URL | https://pubchem.ncbi.nlm.nih.gov | |
Description | Data deposited in or computed by PubChem | |
InChI |
InChI=1S/C29H28O5/c1-4-5-6-7-15-10-16-8-9-18-19(24(16)22(31)11-15)14-21-26(27(18)33)28(34)25-20(29(21,2)3)12-17(30)13-23(25)32/h8-14,30-33H,4-7H2,1-3H3 | |
Source | PubChem | |
URL | https://pubchem.ncbi.nlm.nih.gov | |
Description | Data deposited in or computed by PubChem | |
InChI Key |
QUNHGOCXEGJZAT-UHFFFAOYSA-N | |
Source | PubChem | |
URL | https://pubchem.ncbi.nlm.nih.gov | |
Description | Data deposited in or computed by PubChem | |
Canonical SMILES |
CCCCCC1=CC(=C2C(=C1)C=CC3=C(C4=C(C=C32)C(C5=C(C4=O)C(=CC(=C5)O)O)(C)C)O)O | |
Source | PubChem | |
URL | https://pubchem.ncbi.nlm.nih.gov | |
Description | Data deposited in or computed by PubChem | |
Molecular Formula |
C29H28O5 | |
Source | PubChem | |
URL | https://pubchem.ncbi.nlm.nih.gov | |
Description | Data deposited in or computed by PubChem | |
DSSTOX Substance ID |
DTXSID10164480 | |
Record name | Benastatin C | |
Source | EPA DSSTox | |
URL | https://comptox.epa.gov/dashboard/DTXSID10164480 | |
Description | DSSTox provides a high quality public chemistry resource for supporting improved predictive toxicology. | |
Molecular Weight |
456.5 g/mol | |
Source | PubChem | |
URL | https://pubchem.ncbi.nlm.nih.gov | |
Description | Data deposited in or computed by PubChem | |
CAS No. |
150151-88-5 | |
Record name | Benastatin C | |
Source | ChemIDplus | |
URL | https://pubchem.ncbi.nlm.nih.gov/substance/?source=chemidplus&sourceid=0150151885 | |
Description | ChemIDplus is a free, web search system that provides access to the structure and nomenclature authority files used for the identification of chemical substances cited in National Library of Medicine (NLM) databases, including the TOXNET system. | |
Record name | Benastatin C | |
Source | EPA DSSTox | |
URL | https://comptox.epa.gov/dashboard/DTXSID10164480 | |
Description | DSSTox provides a high quality public chemistry resource for supporting improved predictive toxicology. | |
Retrosynthesis Analysis
AI-Powered Synthesis Planning: Our tool employs the Template_relevance Pistachio, Template_relevance Bkms_metabolic, Template_relevance Pistachio_ringbreaker, Template_relevance Reaxys, Template_relevance Reaxys_biocatalysis model, leveraging a vast database of chemical reactions to predict feasible synthetic routes.
One-Step Synthesis Focus: Specifically designed for one-step synthesis, it provides concise and direct routes for your target compounds, streamlining the synthesis process.
Accurate Predictions: Utilizing the extensive PISTACHIO, BKMS_METABOLIC, PISTACHIO_RINGBREAKER, REAXYS, REAXYS_BIOCATALYSIS database, our tool offers high-accuracy predictions, reflecting the latest in chemical research and data.
Strategy Settings
Precursor scoring | Relevance Heuristic |
---|---|
Min. plausibility | 0.01 |
Model | Template_relevance |
Template Set | Pistachio/Bkms_metabolic/Pistachio_ringbreaker/Reaxys/Reaxys_biocatalysis |
Top-N result to add to graph | 6 |
Feasible Synthetic Routes
Q1: What is the structural relationship between Benastatin C and other members of the Benastatin family, and how does this relate to their activity?
A1: this compound is structurally similar to Benastatin A, differing only by the absence of a carboxyl group at the 2-position (2-decarboxy-Benastatin A) []. This structural difference is significant as it highlights the importance of the carboxyl group for the activity of Benastatin A. While both this compound and D (2-decarboxy-Benastatin B) exhibit inhibitory activity against GST pi and stimulatory activity on murine lymphocyte blastogenesis, further research is needed to determine if the potency or selectivity of these compounds differ from their carboxylated counterparts [].
試験管内研究製品の免責事項と情報
BenchChemで提示されるすべての記事および製品情報は、情報提供を目的としています。BenchChemで購入可能な製品は、生体外研究のために特別に設計されています。生体外研究は、ラテン語の "in glass" に由来し、生物体の外で行われる実験を指します。これらの製品は医薬品または薬として分類されておらず、FDAから任何の医療状態、病気、または疾患の予防、治療、または治癒のために承認されていません。これらの製品を人間または動物に体内に導入する形態は、法律により厳格に禁止されています。これらのガイドラインに従うことは、研究と実験において法的および倫理的な基準の遵守を確実にするために重要です。