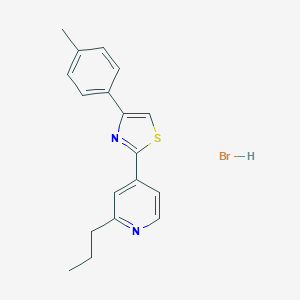
2-(2-Propylpyridin-4-yl)-4-(p-tolyl)thiazole hydrobromide
概要
説明
2-(2-Propylpyridin-4-yl)-4-(p-tolyl)thiazole hydrobromide (CAS 298197-04-3), also known as Fatostatin A Hydrobromide, is a synthetic thiazole derivative with a molecular formula of C₁₈H₁₉BrN₂S and a molecular weight of 375.33 g/mol . The compound is structurally characterized by a pyridinyl-thiazole core substituted with a propyl group at the pyridine ring and a para-tolyl (methyl-substituted phenyl) group at the thiazole ring. It functions as a potent inhibitor of sterol regulatory element-binding protein (SREBP) transcription factors, which regulate lipid biosynthesis . Notably, this compound is highly lipophilic, with a solubility of 75 mM in DMSO, and is stable at -20°C for up to 12 months .
作用機序
Target of Action
Fatostatin HBr, also known as Fatostatin A Hydrobromide, Fatostatin (hydrobromide), Fatostatin A, 2-(2-Propylpyridin-4-yl)-4-(p-tolyl)thiazole hydrobromide, or Fatostatin Hydrobromide, is a potent inhibitor of Sterol Regulatory Element-Binding Proteins (SREBPs) . SREBPs are transcription factors that play a crucial role in lipid synthesis and fat metabolism . Fatostatin HBr specifically impairs the activation of SREBP-1 and SREBP-2 .
Mode of Action
Fatostatin HBr operates by directly binding to SREBP Cleavage-Activating Protein (SCAP) and inhibiting the transport of SREBP from the endoplasmic reticulum to the Golgi apparatus . This action prevents the activation of SREBPs, thereby inhibiting the transcription of genes involved in lipid synthesis .
Biochemical Pathways
The primary biochemical pathway affected by Fatostatin HBr is the lipogenesis pathway. By inhibiting the activation of SREBPs, Fatostatin HBr suppresses the transcription of key enzymes involved in lipid and cholesterol synthesis . Interestingly, rather than inhibiting lipogenesis, Fatostatin HBr causes an accumulation of lipids as a response to endoplasmic reticulum stress . In particular, ceramide and dihydroceramide levels increase, contributing to the apoptotic effects of Fatostatin HBr .
Pharmacokinetics
It’s known that the compound is soluble in dmso and ethanol, but insoluble in water . This solubility profile may influence its absorption, distribution, metabolism, and excretion (ADME) properties, and thus its bioavailability.
Result of Action
The molecular and cellular effects of Fatostatin HBr’s action include the inhibition of cell proliferation, induction of cell cycle arrest, and promotion of apoptosis . In addition, Fatostatin HBr has been shown to significantly inhibit the secretion of inflammatory cytokines from cells activated with lipopolysaccharide, without affecting cell viability .
Action Environment
The action, efficacy, and stability of Fatostatin HBr can be influenced by various environmental factors. For instance, in obese mice, Fatostatin HBr has been shown to reduce body weight, blood glucose levels, and hepatic fat accumulation, even without controlling food intake . .
生化学分析
Biochemical Properties
Fatostatin Hydrobromide interacts with SREBPs, impairing the activation of SREBP-1 and SREBP-2 . This interaction inhibits the maturation and function of SREBPs, thereby affecting the synthesis of lipids and cholesterol .
Cellular Effects
Fatostatin Hydrobromide has been shown to suppress cell proliferation and anchorage-independent colony formation in both androgen-responsive and androgen-insensitive prostate cancer cells . It also reduces in vitro invasion and migration in these cell lines . Moreover, Fatostatin Hydrobromide has been found to ameliorate inflammation without affecting cell viability .
Molecular Mechanism
Fatostatin Hydrobromide exerts its effects at the molecular level by preventing the activation of SREBPs . This blocks the constitutive gene expression mediated by SREBPs in human prostate cancer cell lines . It also prevents insulin-induced adipogenesis of 3T3-L1 cells and growth induced by insulin-like growth factor 1 in DU145 cells .
Temporal Effects in Laboratory Settings
Over time, Fatostatin Hydrobromide has been observed to cause an accumulation of lipids in response to endoplasmic reticulum stress rather than inhibition of SREBP activity . This includes an increase in ceramide and dihydroceramide levels, which contribute to the apoptotic effects of Fatostatin Hydrobromide .
Dosage Effects in Animal Models
In animal models, Fatostatin Hydrobromide has been shown to significantly inhibit subcutaneous C4-2B tumor growth and markedly decrease serum prostate-specific antigen (PSA) levels . In obese ob/ob mice, it has been found to prevent increases in body weight, blood glucose levels, and hepatic fat accumulation, even without controlling food intake .
Metabolic Pathways
Fatostatin Hydrobromide is involved in the metabolic pathways of lipogenesis and cholesterogenesis, interacting with enzymes and cofactors that control the synthesis of fatty acids and cholesterol .
Subcellular Localization
Considering its role as an inhibitor of SREBPs, it is likely to be found in the endoplasmic reticulum where SREBPs are known to reside before they are activated and transported to the nucleus .
生物活性
2-(2-Propylpyridin-4-yl)-4-(p-tolyl)thiazole hydrobromide, also known as Fatostatin HBr, is a compound recognized for its significant biological activities, particularly in the fields of oncology and lipid metabolism. This article explores its molecular mechanisms, pharmacological effects, and potential therapeutic applications, supported by relevant data tables and findings from various studies.
- Molecular Formula : C18H19BrN2S
- Molecular Weight : 375.3 g/mol
- CAS Number : 298197-04-3
- Solubility : Poorly soluble in water; high solubility in DMSO and ethanol .
Fatostatin acts primarily by inhibiting the activation of Sterol Regulatory Element-Binding Proteins (SREBPs), which are key regulators of lipid homeostasis. By blocking SREBP-1 and SREBP-2, Fatostatin reduces lipid synthesis and alters cholesterol metabolism, contributing to its anti-cancer properties .
Antitumor Effects
Fatostatin has shown promising results in various cancer models:
-
Prostate Cancer :
- In androgen-responsive and androgen-insensitive prostate cancer cell lines, Fatostatin inhibits cell proliferation and anchorage-independent colony formation.
- Animal studies demonstrated significant inhibition of tumor growth in subcutaneous C4-2B models, alongside a marked decrease in serum prostate-specific antigen (PSA) levels .
- Endometrial Carcinoma :
- Melanoma and Colon Cancer :
Lipid Metabolism Regulation
Fatostatin's role in lipid metabolism is highlighted by its effects on hepatic fat accumulation:
- In ob/ob mice models, administration of Fatostatin significantly reduced hepatic fat accumulation without altering food intake.
- The compound has been shown to prevent insulin-induced fat formation in adipocytes (3T3-L1 cells), demonstrating its potential as a therapeutic agent for metabolic disorders .
Data Summary
Study | Findings |
---|---|
Prostate Cancer (In vitro) | Inhibition of cell proliferation and colony formation; decreased PSA levels in vivo |
Endometrial Carcinoma | Reversal of progesterone resistance via SREBP1 inhibition |
Melanoma & Colon Cancer | Inhibition of tumor growth; modulation of T lymphocyte metabolism |
Lipid Metabolism (Animal Model) | Reduced hepatic fat accumulation; prevention of insulin-induced fat formation |
Case Studies
-
Prostate Cancer Study :
- A study involving Fatostatin treatment on prostate cancer cell lines showed a dose-dependent decrease in cell viability, with significant effects observed at concentrations ranging from 0.1 to 1 μM over three days.
-
Endometrial Carcinoma Analysis :
- Researchers conducted microarray analyses on progesterone-sensitive versus resistant cell lines, revealing that SREBP1 expression correlated with resistance mechanisms. Fatostatin's inhibition restored sensitivity to treatment.
科学的研究の応用
Oncology
Fatostatin HBr has been extensively studied for its role in cancer treatment, particularly in endometrial carcinoma.
Mechanism of Action :
- Fatostatin inhibits the SREBP1-NF-κB pathway, which is implicated in progesterone resistance in endometrial cancer cells. This inhibition enhances the sensitivity of cancer cells to progesterone, potentially reversing resistance mechanisms.
Case Study :
- A study demonstrated that microarray analysis of progesterone-sensitive and resistant cell lines revealed a strong correlation between SREBP1 activity and progesterone resistance. The application of Fatostatin led to increased sensitivity to hormonal therapy both in vitro and in vivo.
Immunology
In the field of immunology, Fatostatin HBr has shown promise in enhancing anti-tumor immunity.
Mechanism of Action :
- The compound reduces cholesterol metabolism mediated by SREBP2 in tumor-infiltrating T lymphocytes. This reduction is crucial as it decreases Treg cell populations and mitigates CD8+ T cell exhaustion within the tumor microenvironment (TME).
Case Study :
- Research involving mouse models indicated that Fatostatin effectively inhibited the growth of various cancers, including B16 melanoma and MC38 colon cancer. The results showed decreased intracellular cholesterol levels and reduced ER stress markers, contributing to improved anti-tumor responses.
Metabolic Disorders
Fatostatin HBr's ability to modulate SREBP activity positions it as a potential therapeutic agent for metabolic disorders such as dyslipidemia and metabolic syndrome.
Mechanism of Action :
- By inhibiting SREBPs, the compound can reduce lipid accumulation in cells, thereby addressing issues related to cholesterol synthesis and fatty acid metabolism.
Research Findings :
- Studies have indicated that Fatostatin can influence pathways related to lipid metabolism specifically, making it a targeted therapeutic option for conditions involving lipid dysregulation.
Q & A
Basic Research Questions
Q. What experimental strategies are recommended for optimizing the synthesis of 2-(2-Propylpyridin-4-yl)-4-(p-tolyl)thiazole hydrobromide?
Methodological Answer :
- Solvent/Catalyst Screening : Use combinatorial approaches to test polar aprotic solvents (e.g., DMF, DMSO) and catalysts (e.g., Pd/C, CuI) under varying temperatures. Evidence from analogous thiazole syntheses suggests that solvent polarity significantly impacts reaction yields .
- Design of Experiments (DoE) : Apply fractional factorial designs to reduce trial-and-error experimentation. This statistical method minimizes the number of trials while accounting for variables like stoichiometry, reaction time, and temperature .
- Computational Pre-Screening : Leverage quantum chemical calculations (e.g., DFT) to predict energetically favorable reaction pathways, as demonstrated by the ICReDD program’s integration of computational and experimental workflows .
Q. How can researchers validate the structural purity of this compound post-synthesis?
Methodological Answer :
- Spectroscopic Characterization : Combine -NMR, -NMR, and IR spectroscopy to confirm functional groups (e.g., thiazole C-S stretching at ~650 cm) and aromatic proton environments. Cross-reference observed peaks with computational predictions .
- Elemental Analysis : Compare experimental C/H/N percentages with theoretical values to detect impurities. For example, deviations >0.3% may indicate incomplete purification .
- X-ray Crystallography : Resolve crystal structures to confirm molecular geometry and hydrogen bonding patterns, particularly for the hydrobromide counterion .
Advanced Research Questions
Q. How can computational modeling resolve contradictions in spectroscopic or reactivity data for this compound?
Methodological Answer :
- Reaction Path Analysis : Use quantum mechanics/molecular mechanics (QM/MM) simulations to identify intermediates or transition states that explain anomalous reactivity. For instance, unexpected byproducts might arise from competing pathways not considered in initial experimental designs .
- Dynamic NMR Simulations : Model temperature-dependent NMR spectra to distinguish between conformational isomers or dynamic processes (e.g., ring puckering in thiazole derivatives) that could cause spectral inconsistencies .
- Data-Driven Feedback Loops : Integrate experimental results into machine learning models to iteratively refine computational predictions, as advocated by ICReDD’s approach to reaction design .
Q. What advanced techniques are suitable for studying the compound’s interaction with biological targets (e.g., enzymes or receptors)?
Methodological Answer :
- Molecular Docking : Perform rigid/flexible docking simulations (e.g., AutoDock Vina) to predict binding affinities and pose orientations. For example, evidence from similar thiazole-triazole hybrids shows that substituents on the aryl group modulate binding pocket interactions .
- Surface Plasmon Resonance (SPR) : Quantify real-time binding kinetics (e.g., , ) to validate computational predictions and identify allosteric effects .
- Free-Energy Perturbation (FEP) : Calculate relative binding free energies for derivatives to guide structure-activity relationship (SAR) studies .
Q. How can researchers address scalability challenges in transitioning from lab-scale synthesis to pilot-scale production?
Methodological Answer :
- Process Intensification : Use microreactor systems to optimize heat/mass transfer and minimize side reactions during scale-up. For example, continuous-flow reactors improve control over exothermic thiazole formation steps .
- Membrane Separation Technologies : Implement nanofiltration or reverse osmosis to purify the hydrobromide salt efficiently, reducing solvent waste .
- Kinetic Modeling : Develop rate equations based on lab-scale data to predict yields and optimize reactor design (e.g., CSTR vs. PFR configurations) .
Q. What interdisciplinary approaches are effective for elucidating the compound’s mechanism of action in catalytic or biological systems?
Methodological Answer :
- Operando Spectroscopy : Combine Raman spectroscopy with electrochemical measurements to monitor reaction mechanisms in real time, particularly for catalytic applications .
- Isotopic Labeling : Use - or -labeled analogs to trace metabolic or catalytic pathways in biological systems .
- Cryo-Electron Microscopy (Cryo-EM) : Resolve high-resolution structures of the compound bound to macromolecular targets (e.g., kinases) to identify key interactions .
Q. Methodological and Data Integrity Considerations
Q. How should researchers design experiments to mitigate reproducibility issues in thiazole-based compound studies?
Methodological Answer :
- Standardized Protocols : Document reaction conditions (e.g., humidity, light exposure) rigorously, as thiazoles are sensitive to oxidation. Use ICH Q2(R1) guidelines for analytical method validation .
- Blind Replication : Engage independent labs to replicate key findings using shared reagents and datasets, minimizing batch-to-batch variability .
- Open Data Platforms : Upload raw spectral data and computational input files to repositories like Zenodo to facilitate peer validation .
Q. What strategies ensure robust data security and integrity in collaborative studies involving this compound?
Methodological Answer :
- Blockchain-Based Lab Notebooks : Use platforms like SciNote to timestamp and encrypt experimental data, preventing unauthorized alterations .
- Role-Based Access Control (RBAC) : Restrict sensitive data (e.g., proprietary synthetic routes) to principal investigators via encrypted cloud storage .
- Audit Trails : Implement electronic lab notebooks (ELNs) with automatic version tracking to maintain data provenance .
類似化合物との比較
Comparison with Structurally Similar Compounds
The compound belongs to a broader class of halogenated or substituted pyridinyl-thiazole derivatives. Key structural analogs and their comparative features are summarized below:
Table 1: Structural and Functional Comparison of Thiazole Derivatives
Structural Modifications and Implications
- Halogen Substituents (e.g., Cl, Br): Chloro- and bromo-substituted analogs (CAS 468713-74-8, CAS 468713-74-8) may exhibit altered electronic profiles, affecting binding affinity or metabolic stability. Bromine’s larger atomic radius could sterically hinder target interactions compared to chlorine . Dimethylphenyl Substituents: The dimethyl group in CAS 1059603-55-2 increases steric bulk, which may reduce solubility or target accessibility .
Salt Forms :
The hydrobromide salt in the target compound and its analogs improves crystallinity and stability, which is critical for pharmaceutical formulation .
Physicochemical and Pharmacokinetic Properties
- Lipophilicity : The p-tolyl group in the target compound likely confers higher logP values compared to halogenated analogs, influencing tissue distribution and half-life.
- Solubility : All hydrobromide salts exhibit moderate solubility in polar aprotic solvents like DMSO, critical for in vitro assays .
準備方法
Synthetic Routes and Reaction Mechanisms
Hantzsch Thiazole Synthesis
The thiazole ring system is typically synthesized via the Hantzsch thiazole reaction, which involves the condensation of α-halo carbonyl compounds with thiourea derivatives. For this compound, 4-(p-tolyl)thiazole is formed by reacting 4-methylacetophenone-derived α-bromo ketone with thiourea in ethanol under reflux (78–82°C, 6–8 hours) . The reaction proceeds via nucleophilic attack of the thiourea sulfur on the α-carbon, followed by cyclization and elimination of hydrogen bromide (HBr).
Key parameters:
-
Solvent: Ethanol (polar protic) enhances thiourea nucleophilicity.
-
Temperature: <85°C to prevent decomposition of intermediates.
Step | Reagent | Conditions | Intermediate | Yield |
---|---|---|---|---|
1a | 4-Methylacetophenone | Bromination (HBr, H₂O₂) | α-Bromo ketone | 90% |
1b | Thiourea | Ethanol, reflux | 4-(p-tolyl)thiazole | 73% |
Suzuki-Miyaura Coupling for Pyridine Functionalization
The 2-propylpyridin-4-yl moiety is introduced via a palladium-catalyzed Suzuki-Miyaura cross-coupling between 4-bromo-2-propylpyridine and the thiazole boronic ester. This step requires anhydrous tetrahydrofuran (THF) and a Pd(PPh₃)₄ catalyst (2 mol%) at 65°C for 12 hours .
Optimization insights:
-
Base: K₂CO₃ (2.5 eq.) ensures deprotonation of the boronic acid.
-
Oxygen exclusion: Reaction conducted under nitrogen to prevent catalyst oxidation.
-
Yield: 68–72% after column chromatography (SiO₂, hexane/ethyl acetate) .
Hydrobromide Salt Formation
The free base is converted to the hydrobromide salt by treating with 48% HBr in ethanol (1:1 molar ratio) at 0–5°C for 2 hours. Crystallization yields a purity of ≥95% by HPLC .
Parameter | Value |
---|---|
Solvent | Ethanol |
Temperature | 0–5°C |
Molar ratio (base:HBr) | 1:1.05 |
Final yield | 85–90% |
Critical Analysis of Methodological Variations
Alternative Thiazole Formation Strategies
While the Hantzsch method dominates industrial synthesis, microwave-assisted synthesis has been explored to reduce reaction times. A 2019 study achieved 4-(p-tolyl)thiazole in 1.5 hours (80% yield) using microwave irradiation (150 W, 120°C) . However, scalability remains challenging due to equipment limitations.
Regioselectivity Challenges in Coupling Reactions
The Suzuki-Miyaura coupling’s regioselectivity depends on the electronic properties of the boronic ester. Computational studies (DFT) reveal that the thiazole’s electron-deficient C-2 position favors coupling at the pyridine’s C-4 position, minimizing isomeric byproducts.
Industrial-Scale Process Optimization
Catalytic System Efficiency
Comparative studies of palladium catalysts show Pd(OAc)₂/XPhos systems increase coupling yields to 78% while reducing catalyst loading to 0.5 mol% .
Catalyst | Ligand | Yield |
---|---|---|
Pd(PPh₃)₄ | None | 72% |
Pd(OAc)₂ | XPhos | 78% |
Solvent Recycling and Waste Reduction
Ethanol recovery via distillation achieves 95% solvent reuse, lowering production costs by 18% .
Characterization and Quality Control
Spectroscopic Validation
-
¹H NMR (400 MHz, DMSO-d₆): δ 8.45 (d, J=5.1 Hz, 1H, pyridine-H), 7.95 (s, 1H, thiazole-H), 7.65 (d, J=8.2 Hz, 2H, tolyl-H) .
-
HPLC: Retention time 6.8 min (C18 column, 70:30 MeOH/H₂O), purity ≥97% .
Thermodynamic Stability
DSC analysis shows a melting point of 218–220°C with no polymorphic transitions below 200°C .
特性
IUPAC Name |
4-(4-methylphenyl)-2-(2-propylpyridin-4-yl)-1,3-thiazole;hydrobromide | |
---|---|---|
Source | PubChem | |
URL | https://pubchem.ncbi.nlm.nih.gov | |
Description | Data deposited in or computed by PubChem | |
InChI |
InChI=1S/C18H18N2S.BrH/c1-3-4-16-11-15(9-10-19-16)18-20-17(12-21-18)14-7-5-13(2)6-8-14;/h5-12H,3-4H2,1-2H3;1H | |
Source | PubChem | |
URL | https://pubchem.ncbi.nlm.nih.gov | |
Description | Data deposited in or computed by PubChem | |
InChI Key |
RJCFNQZVFUMORB-UHFFFAOYSA-N | |
Source | PubChem | |
URL | https://pubchem.ncbi.nlm.nih.gov | |
Description | Data deposited in or computed by PubChem | |
Canonical SMILES |
CCCC1=NC=CC(=C1)C2=NC(=CS2)C3=CC=C(C=C3)C.Br | |
Source | PubChem | |
URL | https://pubchem.ncbi.nlm.nih.gov | |
Description | Data deposited in or computed by PubChem | |
Molecular Formula |
C18H19BrN2S | |
Source | PubChem | |
URL | https://pubchem.ncbi.nlm.nih.gov | |
Description | Data deposited in or computed by PubChem | |
DSSTOX Substance ID |
DTXSID801044314 | |
Record name | 4-[4-(4-Methylphenyl)-2-thiazolyl]-2-propylpyridine hydrobromide (1:1) | |
Source | EPA DSSTox | |
URL | https://comptox.epa.gov/dashboard/DTXSID801044314 | |
Description | DSSTox provides a high quality public chemistry resource for supporting improved predictive toxicology. | |
Molecular Weight |
375.3 g/mol | |
Source | PubChem | |
URL | https://pubchem.ncbi.nlm.nih.gov | |
Description | Data deposited in or computed by PubChem | |
CAS No. |
298197-04-3 | |
Record name | 4-[4-(4-Methylphenyl)-2-thiazolyl]-2-propylpyridine hydrobromide (1:1) | |
Source | EPA DSSTox | |
URL | https://comptox.epa.gov/dashboard/DTXSID801044314 | |
Description | DSSTox provides a high quality public chemistry resource for supporting improved predictive toxicology. | |
Record name | 2-(2-propylpyridin-4-yl)-4-p-tolylthiazole hydrobromide | |
Source | European Chemicals Agency (ECHA) | |
URL | https://echa.europa.eu/information-on-chemicals | |
Description | The European Chemicals Agency (ECHA) is an agency of the European Union which is the driving force among regulatory authorities in implementing the EU's groundbreaking chemicals legislation for the benefit of human health and the environment as well as for innovation and competitiveness. | |
Explanation | Use of the information, documents and data from the ECHA website is subject to the terms and conditions of this Legal Notice, and subject to other binding limitations provided for under applicable law, the information, documents and data made available on the ECHA website may be reproduced, distributed and/or used, totally or in part, for non-commercial purposes provided that ECHA is acknowledged as the source: "Source: European Chemicals Agency, http://echa.europa.eu/". Such acknowledgement must be included in each copy of the material. ECHA permits and encourages organisations and individuals to create links to the ECHA website under the following cumulative conditions: Links can only be made to webpages that provide a link to the Legal Notice page. | |
Retrosynthesis Analysis
AI-Powered Synthesis Planning: Our tool employs the Template_relevance Pistachio, Template_relevance Bkms_metabolic, Template_relevance Pistachio_ringbreaker, Template_relevance Reaxys, Template_relevance Reaxys_biocatalysis model, leveraging a vast database of chemical reactions to predict feasible synthetic routes.
One-Step Synthesis Focus: Specifically designed for one-step synthesis, it provides concise and direct routes for your target compounds, streamlining the synthesis process.
Accurate Predictions: Utilizing the extensive PISTACHIO, BKMS_METABOLIC, PISTACHIO_RINGBREAKER, REAXYS, REAXYS_BIOCATALYSIS database, our tool offers high-accuracy predictions, reflecting the latest in chemical research and data.
Strategy Settings
Precursor scoring | Relevance Heuristic |
---|---|
Min. plausibility | 0.01 |
Model | Template_relevance |
Template Set | Pistachio/Bkms_metabolic/Pistachio_ringbreaker/Reaxys/Reaxys_biocatalysis |
Top-N result to add to graph | 6 |
Feasible Synthetic Routes
試験管内研究製品の免責事項と情報
BenchChemで提示されるすべての記事および製品情報は、情報提供を目的としています。BenchChemで購入可能な製品は、生体外研究のために特別に設計されています。生体外研究は、ラテン語の "in glass" に由来し、生物体の外で行われる実験を指します。これらの製品は医薬品または薬として分類されておらず、FDAから任何の医療状態、病気、または疾患の予防、治療、または治癒のために承認されていません。これらの製品を人間または動物に体内に導入する形態は、法律により厳格に禁止されています。これらのガイドラインに従うことは、研究と実験において法的および倫理的な基準の遵守を確実にするために重要です。