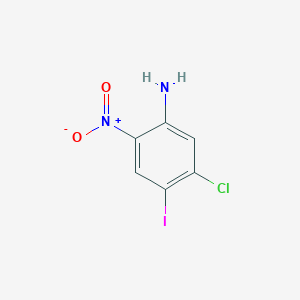
5-クロロ-4-ヨード-2-ニトロアニリン
概要
説明
5-Chloro-4-iodo-2-nitroaniline: is an organic compound with the molecular formula C6H4ClIN2O2 It is a derivative of aniline, where the hydrogen atoms on the benzene ring are substituted with chlorine, iodine, and nitro groups
科学的研究の応用
Chemistry:
Intermediate in Organic Synthesis: 5-Chloro-4-iodo-2-nitroaniline is used as an intermediate in the synthesis of more complex organic molecules.
Material Science: It is used in the development of high refractive index materials and polymers.
Biology and Medicine:
Pharmaceutical Research: The compound is investigated for its potential use in drug development due to its unique structural properties.
Industry:
Dye and Pigment Production: It is used in the synthesis of dyes and pigments due to its chromophoric properties.
作用機序
Target of Action
Nitro compounds, in general, are known to interact with a variety of biological targets, including enzymes and cellular structures .
Mode of Action
Nitro compounds typically undergo reduction to form reactive intermediates, which can interact with cellular targets . The iodine and chlorine substituents may also influence the compound’s reactivity and interactions with its targets.
Biochemical Pathways
Nitro compounds can participate in various biochemical reactions, including redox reactions and electrophilic substitutions .
Pharmacokinetics
It is suggested that the compound has high gastrointestinal absorption and is bbb permeant . The compound is predicted to have moderate water solubility .
Result of Action
Nitro compounds can cause a variety of cellular effects, depending on their specific targets and mode of action .
Action Environment
Environmental factors can significantly influence the action, efficacy, and stability of 5-Chloro-4-iodo-2-nitroaniline. For instance, the compound should be stored in a dark place, sealed in dry conditions, and at a temperature of 2-8°C to maintain its stability . The compound’s action and efficacy could also be influenced by factors such as pH, temperature, and the presence of other substances in the environment.
生化学分析
Biochemical Properties
5-Chloro-4-iodo-2-nitroaniline plays a significant role in biochemical reactions. It interacts with various enzymes, proteins, and other biomolecules. For instance, it has been identified as an inhibitor of certain cytochrome P450 enzymes, including CYP2C19 and CYP2C9 . These interactions are crucial as they can affect the metabolism of other compounds and drugs within the body. The inhibitory action of 5-Chloro-4-iodo-2-nitroaniline on these enzymes can lead to altered metabolic pathways and potential drug-drug interactions.
Cellular Effects
The effects of 5-Chloro-4-iodo-2-nitroaniline on cellular processes are diverse. It has been observed to influence cell signaling pathways, gene expression, and cellular metabolism. For example, its interaction with cytochrome P450 enzymes can lead to changes in the expression of genes involved in metabolic processes . Additionally, 5-Chloro-4-iodo-2-nitroaniline can affect cellular metabolism by altering the activity of enzymes that play a role in metabolic pathways, potentially leading to changes in the levels of various metabolites.
Molecular Mechanism
At the molecular level, 5-Chloro-4-iodo-2-nitroaniline exerts its effects through binding interactions with biomolecules. It binds to the active sites of cytochrome P450 enzymes, inhibiting their activity . This inhibition can lead to a decrease in the metabolism of substrates that are normally processed by these enzymes. Furthermore, 5-Chloro-4-iodo-2-nitroaniline can induce changes in gene expression by affecting transcription factors and other regulatory proteins involved in gene regulation.
Temporal Effects in Laboratory Settings
In laboratory settings, the effects of 5-Chloro-4-iodo-2-nitroaniline can change over time. The compound is relatively stable when stored in a dark, dry place at temperatures between 2-8°C . Its stability can be affected by exposure to light and moisture, leading to degradation. Long-term studies have shown that 5-Chloro-4-iodo-2-nitroaniline can have sustained effects on cellular function, particularly in in vitro studies where it continues to inhibit enzyme activity over extended periods.
Dosage Effects in Animal Models
The effects of 5-Chloro-4-iodo-2-nitroaniline vary with different dosages in animal models. At lower doses, it can effectively inhibit cytochrome P450 enzymes without causing significant toxicity . At higher doses, it may lead to toxic effects, including liver damage and disruption of normal metabolic processes. These adverse effects highlight the importance of careful dosage control in experimental settings.
Metabolic Pathways
5-Chloro-4-iodo-2-nitroaniline is involved in several metabolic pathways. It interacts with enzymes such as cytochrome P450, which are crucial for the metabolism of various endogenous and exogenous compounds . The inhibition of these enzymes by 5-Chloro-4-iodo-2-nitroaniline can lead to changes in metabolic flux and the accumulation of certain metabolites. This can have downstream effects on cellular function and overall metabolism.
Transport and Distribution
Within cells and tissues, 5-Chloro-4-iodo-2-nitroaniline is transported and distributed through interactions with transporters and binding proteins . These interactions facilitate its localization to specific cellular compartments where it can exert its effects. The compound’s distribution is influenced by its physicochemical properties, including its lipophilicity and solubility.
Subcellular Localization
The subcellular localization of 5-Chloro-4-iodo-2-nitroaniline is critical for its activity and function. It is directed to specific compartments within the cell, such as the endoplasmic reticulum, where cytochrome P450 enzymes are located . This targeting is facilitated by post-translational modifications and specific targeting signals that ensure the compound reaches its site of action.
準備方法
Synthetic Routes and Reaction Conditions:
Starting Materials: The synthesis of 5-Chloro-4-iodo-2-nitroaniline typically begins with commercially available starting materials such as 2,4-dichloronitrobenzene.
Iodination: The final step involves the iodination of the intermediate compound using iodine and a suitable catalyst.
Industrial Production Methods: Industrial production methods for 5-Chloro-4-iodo-2-nitroaniline involve similar synthetic routes but are optimized for large-scale production. These methods emphasize high yield, purity, and cost-effectiveness. The use of continuous flow reactors and advanced purification techniques ensures the efficient production of this compound.
化学反応の分析
Types of Reactions:
Electrophilic Aromatic Substitution: The compound can undergo electrophilic aromatic substitution reactions due to the presence of electron-withdrawing groups like nitro and halogens.
Nucleophilic Aromatic Substitution: The compound can participate in nucleophilic aromatic substitution reactions, especially when activated by electron-withdrawing groups.
Common Reagents and Conditions:
Electrophilic Aromatic Substitution: Reagents like chlorine, bromine, and sulfuric acid are commonly used.
Reduction: Hydrogen gas with a palladium catalyst or sodium borohydride.
Nucleophilic Aromatic Substitution: Strong nucleophiles like dimethylamine in ethanol.
Major Products:
Reduction: 5-Chloro-4-iodo-2-aminoaniline.
Substitution: Various substituted anilines depending on the nucleophile used.
類似化合物との比較
2-Chloro-4-nitroaniline: Similar in structure but lacks the iodine atom.
4-Iodo-2-nitroaniline: Similar but lacks the chlorine atom.
Uniqueness:
Halogen Substitution: The presence of both chlorine and iodine atoms in 5-Chloro-4-iodo-2-nitroaniline makes it unique compared to other nitroanilines
特性
IUPAC Name |
5-chloro-4-iodo-2-nitroaniline | |
---|---|---|
Source | PubChem | |
URL | https://pubchem.ncbi.nlm.nih.gov | |
Description | Data deposited in or computed by PubChem | |
InChI |
InChI=1S/C6H4ClIN2O2/c7-3-1-5(9)6(10(11)12)2-4(3)8/h1-2H,9H2 | |
Source | PubChem | |
URL | https://pubchem.ncbi.nlm.nih.gov | |
Description | Data deposited in or computed by PubChem | |
InChI Key |
KWHURWOGZQWLTK-UHFFFAOYSA-N | |
Source | PubChem | |
URL | https://pubchem.ncbi.nlm.nih.gov | |
Description | Data deposited in or computed by PubChem | |
Canonical SMILES |
C1=C(C(=CC(=C1Cl)I)[N+](=O)[O-])N | |
Source | PubChem | |
URL | https://pubchem.ncbi.nlm.nih.gov | |
Description | Data deposited in or computed by PubChem | |
Molecular Formula |
C6H4ClIN2O2 | |
Source | PubChem | |
URL | https://pubchem.ncbi.nlm.nih.gov | |
Description | Data deposited in or computed by PubChem | |
DSSTOX Substance ID |
DTXSID40624688 | |
Record name | 5-Chloro-4-iodo-2-nitroaniline | |
Source | EPA DSSTox | |
URL | https://comptox.epa.gov/dashboard/DTXSID40624688 | |
Description | DSSTox provides a high quality public chemistry resource for supporting improved predictive toxicology. | |
Molecular Weight |
298.46 g/mol | |
Source | PubChem | |
URL | https://pubchem.ncbi.nlm.nih.gov | |
Description | Data deposited in or computed by PubChem | |
CAS No. |
335349-57-0 | |
Record name | 5-Chloro-4-iodo-2-nitroaniline | |
Source | EPA DSSTox | |
URL | https://comptox.epa.gov/dashboard/DTXSID40624688 | |
Description | DSSTox provides a high quality public chemistry resource for supporting improved predictive toxicology. | |
Synthesis routes and methods I
Procedure details
Synthesis routes and methods II
Procedure details
Synthesis routes and methods III
Procedure details
Retrosynthesis Analysis
AI-Powered Synthesis Planning: Our tool employs the Template_relevance Pistachio, Template_relevance Bkms_metabolic, Template_relevance Pistachio_ringbreaker, Template_relevance Reaxys, Template_relevance Reaxys_biocatalysis model, leveraging a vast database of chemical reactions to predict feasible synthetic routes.
One-Step Synthesis Focus: Specifically designed for one-step synthesis, it provides concise and direct routes for your target compounds, streamlining the synthesis process.
Accurate Predictions: Utilizing the extensive PISTACHIO, BKMS_METABOLIC, PISTACHIO_RINGBREAKER, REAXYS, REAXYS_BIOCATALYSIS database, our tool offers high-accuracy predictions, reflecting the latest in chemical research and data.
Strategy Settings
Precursor scoring | Relevance Heuristic |
---|---|
Min. plausibility | 0.01 |
Model | Template_relevance |
Template Set | Pistachio/Bkms_metabolic/Pistachio_ringbreaker/Reaxys/Reaxys_biocatalysis |
Top-N result to add to graph | 6 |
Feasible Synthetic Routes
Q1: What makes 5-chloro-4-iodo-2-nitroaniline a suitable starting material for benzimidazole synthesis?
A1: The research paper [] highlights that 5-chloro-4-iodo-2-nitroaniline possesses structural features beneficial for generating diverse benzimidazole derivatives. The presence of both chlorine and iodine substituents allows for selective functionalization at these positions. This enables the introduction of various substituents to the benzimidazole core, which is crucial for exploring structure-activity relationships and developing compounds with desired properties. Furthermore, the nitro group can be readily reduced to an amine, providing another handle for further derivatization. This versatility makes 5-chloro-4-iodo-2-nitroaniline a valuable building block in organic synthesis, particularly for generating diverse libraries of benzimidazole compounds.
試験管内研究製品の免責事項と情報
BenchChemで提示されるすべての記事および製品情報は、情報提供を目的としています。BenchChemで購入可能な製品は、生体外研究のために特別に設計されています。生体外研究は、ラテン語の "in glass" に由来し、生物体の外で行われる実験を指します。これらの製品は医薬品または薬として分類されておらず、FDAから任何の医療状態、病気、または疾患の予防、治療、または治癒のために承認されていません。これらの製品を人間または動物に体内に導入する形態は、法律により厳格に禁止されています。これらのガイドラインに従うことは、研究と実験において法的および倫理的な基準の遵守を確実にするために重要です。