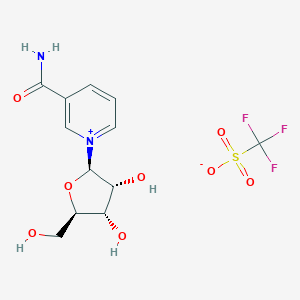
Nicotinamide Riboside Triflate
概要
説明
Nicotinamide Riboside Triflate is a derivative of Nicotinamide Riboside, a form of vitamin B3. It is an intermediate in the biosynthetic pathway by which Nicotinamide is converted into Nicotinamide Adenine Dinucleotide (NAD+), a crucial coenzyme in cellular metabolism . This compound has garnered significant attention due to its potential health benefits and applications in various scientific fields.
科学的研究の応用
Nicotinamide Riboside Triflate has a wide range of applications in scientific research:
Chemistry: It is used as a precursor in the synthesis of various Nicotinamide Riboside derivatives.
Biology: It is studied for its role in cellular metabolism and its potential to enhance NAD+ levels.
作用機序
準備方法
Synthetic Routes and Reaction Conditions: The synthesis of Nicotinamide Riboside Triflate typically involves the triflation of Nicotinamide Riboside. One common method includes the reaction of Nicotinamide Riboside with triflic anhydride in the presence of a base such as pyridine. The reaction is carried out in an anhydrous solvent like dichloromethane at low temperatures to prevent decomposition .
Industrial Production Methods: Industrial production of this compound follows similar synthetic routes but on a larger scale. The process involves careful control of reaction conditions to ensure high yield and purity. The product is then purified using techniques such as column chromatography and recrystallization .
化学反応の分析
Types of Reactions: Nicotinamide Riboside Triflate undergoes various chemical reactions, including:
Substitution Reactions: It can participate in nucleophilic substitution reactions where the triflate group is replaced by other nucleophiles.
Reduction Reactions: It can be reduced to form Nicotinamide Riboside.
Common Reagents and Conditions:
Substitution Reactions: Common reagents include nucleophiles such as amines or thiols. The reactions are typically carried out in polar aprotic solvents like dimethylformamide (DMF) at moderate temperatures.
Reduction Reactions: Reducing agents such as sodium borohydride or lithium aluminum hydride are used under anhydrous conditions.
Major Products:
Substitution Reactions: The major products are substituted Nicotinamide Riboside derivatives.
Reduction Reactions: The major product is Nicotinamide Riboside.
類似化合物との比較
Nicotinamide Riboside Chloride: Another derivative of Nicotinamide Riboside, used as a dietary supplement.
Nicotinamide Mononucleotide: A direct precursor to NAD+, also studied for its potential health benefits.
Uniqueness: Nicotinamide Riboside Triflate is unique due to its triflate group, which makes it a versatile intermediate for further chemical modifications. This allows for the synthesis of a wide range of derivatives with potential therapeutic applications .
特性
IUPAC Name |
1-[(2R,3R,4S,5R)-3,4-dihydroxy-5-(hydroxymethyl)oxolan-2-yl]pyridin-1-ium-3-carboxamide;trifluoromethanesulfonate | |
---|---|---|
Source | PubChem | |
URL | https://pubchem.ncbi.nlm.nih.gov | |
Description | Data deposited in or computed by PubChem | |
InChI |
InChI=1S/C11H14N2O5.CHF3O3S/c12-10(17)6-2-1-3-13(4-6)11-9(16)8(15)7(5-14)18-11;2-1(3,4)8(5,6)7/h1-4,7-9,11,14-16H,5H2,(H-,12,17);(H,5,6,7)/t7-,8-,9-,11-;/m1./s1 | |
Source | PubChem | |
URL | https://pubchem.ncbi.nlm.nih.gov | |
Description | Data deposited in or computed by PubChem | |
InChI Key |
ZEWRNTDQENDMQK-IVOJBTPCSA-N | |
Source | PubChem | |
URL | https://pubchem.ncbi.nlm.nih.gov | |
Description | Data deposited in or computed by PubChem | |
Canonical SMILES |
C1=CC(=C[N+](=C1)C2C(C(C(O2)CO)O)O)C(=O)N.C(F)(F)(F)S(=O)(=O)[O-] | |
Source | PubChem | |
URL | https://pubchem.ncbi.nlm.nih.gov | |
Description | Data deposited in or computed by PubChem | |
Isomeric SMILES |
C1=CC(=C[N+](=C1)[C@H]2[C@@H]([C@@H]([C@H](O2)CO)O)O)C(=O)N.C(F)(F)(F)S(=O)(=O)[O-] | |
Source | PubChem | |
URL | https://pubchem.ncbi.nlm.nih.gov | |
Description | Data deposited in or computed by PubChem | |
Molecular Formula |
C12H15F3N2O8S | |
Source | PubChem | |
URL | https://pubchem.ncbi.nlm.nih.gov | |
Description | Data deposited in or computed by PubChem | |
DSSTOX Substance ID |
DTXSID20462505 | |
Record name | Ribosylnicotinamide Triflate | |
Source | EPA DSSTox | |
URL | https://comptox.epa.gov/dashboard/DTXSID20462505 | |
Description | DSSTox provides a high quality public chemistry resource for supporting improved predictive toxicology. | |
Molecular Weight |
404.32 g/mol | |
Source | PubChem | |
URL | https://pubchem.ncbi.nlm.nih.gov | |
Description | Data deposited in or computed by PubChem | |
CAS No. |
445489-49-6 | |
Record name | Ribosylnicotinamide Triflate | |
Source | EPA DSSTox | |
URL | https://comptox.epa.gov/dashboard/DTXSID20462505 | |
Description | DSSTox provides a high quality public chemistry resource for supporting improved predictive toxicology. | |
Retrosynthesis Analysis
AI-Powered Synthesis Planning: Our tool employs the Template_relevance Pistachio, Template_relevance Bkms_metabolic, Template_relevance Pistachio_ringbreaker, Template_relevance Reaxys, Template_relevance Reaxys_biocatalysis model, leveraging a vast database of chemical reactions to predict feasible synthetic routes.
One-Step Synthesis Focus: Specifically designed for one-step synthesis, it provides concise and direct routes for your target compounds, streamlining the synthesis process.
Accurate Predictions: Utilizing the extensive PISTACHIO, BKMS_METABOLIC, PISTACHIO_RINGBREAKER, REAXYS, REAXYS_BIOCATALYSIS database, our tool offers high-accuracy predictions, reflecting the latest in chemical research and data.
Strategy Settings
Precursor scoring | Relevance Heuristic |
---|---|
Min. plausibility | 0.01 |
Model | Template_relevance |
Template Set | Pistachio/Bkms_metabolic/Pistachio_ringbreaker/Reaxys/Reaxys_biocatalysis |
Top-N result to add to graph | 6 |
Feasible Synthetic Routes
試験管内研究製品の免責事項と情報
BenchChemで提示されるすべての記事および製品情報は、情報提供を目的としています。BenchChemで購入可能な製品は、生体外研究のために特別に設計されています。生体外研究は、ラテン語の "in glass" に由来し、生物体の外で行われる実験を指します。これらの製品は医薬品または薬として分類されておらず、FDAから任何の医療状態、病気、または疾患の予防、治療、または治癒のために承認されていません。これらの製品を人間または動物に体内に導入する形態は、法律により厳格に禁止されています。これらのガイドラインに従うことは、研究と実験において法的および倫理的な基準の遵守を確実にするために重要です。