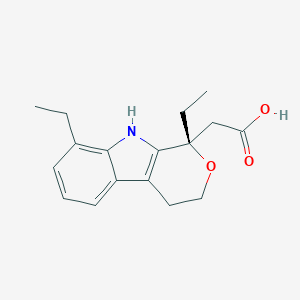
(S)-etodolac
説明
(S)-etodolac is the S-enantiomer of etodolac. It is a preferential inhibitor of cyclo-oxygenase 2 and a non-steroidal anti-inflammatory, whereas the enantiomer, (R)-etodolac, is inactive. The racemate is commonly used for the treatment of rheumatoid arthritis and osteoarthritis, and for the alleviation of postoperative pain. It has a role as a cyclooxygenase 2 inhibitor and a non-narcotic analgesic. It is an enantiomer of a (R)-etodolac.
作用機序
Target of Action
It is known that etodolac, like other non-steroidal anti-inflammatory drugs (nsaids), primarily targets and inhibits cyclooxygenase (cox) enzymes . These enzymes play a crucial role in the synthesis of prostaglandins, which are involved in inflammation and pain signaling in the body .
Mode of Action
(S)-etodolac interacts with its targets, primarily the COX enzymes, by binding to their active sites and inhibiting their activity . This inhibition prevents the conversion of arachidonic acid to prostaglandins, thereby reducing inflammation and pain .
Biochemical Pathways
The key biochemical pathway affected by this compound is the arachidonic acid pathway . By inhibiting COX enzymes, this compound disrupts this pathway, leading to a decrease in the production of prostaglandins. The downstream effect of this disruption is a reduction in inflammation and pain signals .
Pharmacokinetics
The pharmacokinetics of this compound involves its absorption, distribution, metabolism, and excretion (ADME). Generally, NSAIDs like etodolac are well absorbed orally, widely distributed in the body, metabolized in the liver, and excreted in urine . These properties impact the drug’s bioavailability, determining the concentration of the drug that reaches the systemic circulation and its site of action .
Result of Action
The molecular and cellular effects of this compound’s action primarily involve the reduction of prostaglandin synthesis. This leads to decreased inflammation and pain at the cellular level . Additionally, by inhibiting COX enzymes, this compound may also reduce fever and prevent blood clotting, as these enzymes are involved in temperature regulation and platelet aggregation .
Action Environment
Environmental factors can influence the action, efficacy, and stability of this compound. Factors such as pH levels in the stomach and intestines can affect drug absorption, while liver and kidney function can impact drug metabolism and excretion . Furthermore, individual genetic variations can influence how effectively this compound works in different individuals .
特性
IUPAC Name |
2-[(1S)-1,8-diethyl-4,9-dihydro-3H-pyrano[3,4-b]indol-1-yl]acetic acid | |
---|---|---|
Source | PubChem | |
URL | https://pubchem.ncbi.nlm.nih.gov | |
Description | Data deposited in or computed by PubChem | |
InChI |
InChI=1S/C17H21NO3/c1-3-11-6-5-7-12-13-8-9-21-17(4-2,10-14(19)20)16(13)18-15(11)12/h5-7,18H,3-4,8-10H2,1-2H3,(H,19,20)/t17-/m0/s1 | |
Source | PubChem | |
URL | https://pubchem.ncbi.nlm.nih.gov | |
Description | Data deposited in or computed by PubChem | |
InChI Key |
NNYBQONXHNTVIJ-KRWDZBQOSA-N | |
Source | PubChem | |
URL | https://pubchem.ncbi.nlm.nih.gov | |
Description | Data deposited in or computed by PubChem | |
Canonical SMILES |
CCC1=C2C(=CC=C1)C3=C(N2)C(OCC3)(CC)CC(=O)O | |
Source | PubChem | |
URL | https://pubchem.ncbi.nlm.nih.gov | |
Description | Data deposited in or computed by PubChem | |
Isomeric SMILES |
CCC1=C2C(=CC=C1)C3=C(N2)[C@](OCC3)(CC)CC(=O)O | |
Source | PubChem | |
URL | https://pubchem.ncbi.nlm.nih.gov | |
Description | Data deposited in or computed by PubChem | |
Molecular Formula |
C17H21NO3 | |
Source | PubChem | |
URL | https://pubchem.ncbi.nlm.nih.gov | |
Description | Data deposited in or computed by PubChem | |
DSSTOX Substance ID |
DTXSID201043544 | |
Record name | [(1S)-1,8-diethyl-1,3,4,9-tetrahydropyrano[3,4-b]indol-1-yl]acetic acid | |
Source | EPA DSSTox | |
URL | https://comptox.epa.gov/dashboard/DTXSID201043544 | |
Description | DSSTox provides a high quality public chemistry resource for supporting improved predictive toxicology. | |
Molecular Weight |
287.35 g/mol | |
Source | PubChem | |
URL | https://pubchem.ncbi.nlm.nih.gov | |
Description | Data deposited in or computed by PubChem | |
CAS No. |
87249-11-4 | |
Record name | (+)-Etodolac | |
Source | CAS Common Chemistry | |
URL | https://commonchemistry.cas.org/detail?cas_rn=87249-11-4 | |
Description | CAS Common Chemistry is an open community resource for accessing chemical information. Nearly 500,000 chemical substances from CAS REGISTRY cover areas of community interest, including common and frequently regulated chemicals, and those relevant to high school and undergraduate chemistry classes. This chemical information, curated by our expert scientists, is provided in alignment with our mission as a division of the American Chemical Society. | |
Explanation | The data from CAS Common Chemistry is provided under a CC-BY-NC 4.0 license, unless otherwise stated. | |
Record name | Etodolac, (S)- | |
Source | ChemIDplus | |
URL | https://pubchem.ncbi.nlm.nih.gov/substance/?source=chemidplus&sourceid=0087249114 | |
Description | ChemIDplus is a free, web search system that provides access to the structure and nomenclature authority files used for the identification of chemical substances cited in National Library of Medicine (NLM) databases, including the TOXNET system. | |
Record name | [(1S)-1,8-diethyl-1,3,4,9-tetrahydropyrano[3,4-b]indol-1-yl]acetic acid | |
Source | EPA DSSTox | |
URL | https://comptox.epa.gov/dashboard/DTXSID201043544 | |
Description | DSSTox provides a high quality public chemistry resource for supporting improved predictive toxicology. | |
Record name | ETODOLAC, (S)- | |
Source | FDA Global Substance Registration System (GSRS) | |
URL | https://gsrs.ncats.nih.gov/ginas/app/beta/substances/1046G5D6YD | |
Description | The FDA Global Substance Registration System (GSRS) enables the efficient and accurate exchange of information on what substances are in regulated products. Instead of relying on names, which vary across regulatory domains, countries, and regions, the GSRS knowledge base makes it possible for substances to be defined by standardized, scientific descriptions. | |
Explanation | Unless otherwise noted, the contents of the FDA website (www.fda.gov), both text and graphics, are not copyrighted. They are in the public domain and may be republished, reprinted and otherwise used freely by anyone without the need to obtain permission from FDA. Credit to the U.S. Food and Drug Administration as the source is appreciated but not required. | |
Retrosynthesis Analysis
AI-Powered Synthesis Planning: Our tool employs the Template_relevance Pistachio, Template_relevance Bkms_metabolic, Template_relevance Pistachio_ringbreaker, Template_relevance Reaxys, Template_relevance Reaxys_biocatalysis model, leveraging a vast database of chemical reactions to predict feasible synthetic routes.
One-Step Synthesis Focus: Specifically designed for one-step synthesis, it provides concise and direct routes for your target compounds, streamlining the synthesis process.
Accurate Predictions: Utilizing the extensive PISTACHIO, BKMS_METABOLIC, PISTACHIO_RINGBREAKER, REAXYS, REAXYS_BIOCATALYSIS database, our tool offers high-accuracy predictions, reflecting the latest in chemical research and data.
Strategy Settings
Precursor scoring | Relevance Heuristic |
---|---|
Min. plausibility | 0.01 |
Model | Template_relevance |
Template Set | Pistachio/Bkms_metabolic/Pistachio_ringbreaker/Reaxys/Reaxys_biocatalysis |
Top-N result to add to graph | 6 |
Feasible Synthetic Routes
試験管内研究製品の免責事項と情報
BenchChemで提示されるすべての記事および製品情報は、情報提供を目的としています。BenchChemで購入可能な製品は、生体外研究のために特別に設計されています。生体外研究は、ラテン語の "in glass" に由来し、生物体の外で行われる実験を指します。これらの製品は医薬品または薬として分類されておらず、FDAから任何の医療状態、病気、または疾患の予防、治療、または治癒のために承認されていません。これらの製品を人間または動物に体内に導入する形態は、法律により厳格に禁止されています。これらのガイドラインに従うことは、研究と実験において法的および倫理的な基準の遵守を確実にするために重要です。