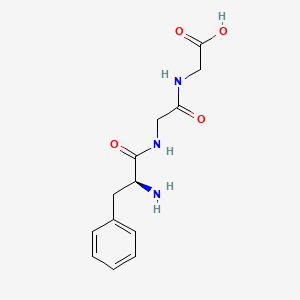
Phe-gly-gly
概要
説明
Phe-gly-gly, also known as phenylalanyl-glycyl-glycine, is a tripeptide composed of the amino acids phenylalanine, glycine, and glycine. Peptides like this compound are short chains of amino acids linked by peptide bonds, which are amide bonds formed between the carboxyl group of one amino acid and the amino group of another. Peptides play crucial roles in various biological processes and have significant applications in scientific research and industry.
作用機序
Target of Action
Phe-gly-gly, a tripeptide composed of one L-phenylalanine and two glycine residues , is known to exhibit significant antioxidant activity . The primary targets of this compound are the reactive oxygen species (ROS) in the body. ROS are chemically reactive molecules that contain oxygen and play crucial roles in cell signaling and homeostasis. Excessive ros can lead to oxidative stress, which is harmful to cells and is linked to various diseases .
Mode of Action
The mode of action of this compound involves its interaction with ROS. The compound’s antioxidant activity allows it to neutralize ROS, thereby preventing oxidative stress . This is achieved through the compound’s ability to donate electrons to ROS, converting them into more stable molecules and preventing them from causing damage to cellular components .
Biochemical Pathways
The antioxidant activity of this compound affects various biochemical pathways related to oxidative stress. By neutralizing ROS, this compound can prevent the oxidative damage to cellular components such as proteins, lipids, and DNA. This can help maintain the integrity of these molecules and ensure the proper functioning of various biochemical processes .
Pharmacokinetics
It is known that peptides can be absorbed in the gastrointestinal tract and distributed throughout the body . The metabolism and excretion of this compound would likely involve enzymatic breakdown and renal excretion, respectively .
Result of Action
The result of this compound’s action is the reduction of oxidative stress in the body. By neutralizing ROS, this compound can prevent oxidative damage to cellular components, thereby maintaining cellular integrity and function . This can have various beneficial effects, including the prevention of diseases associated with oxidative stress .
Action Environment
The action of this compound can be influenced by various environmental factors. For example, the presence of other antioxidants can enhance the compound’s antioxidant activity. Additionally, factors such as pH and temperature can affect the stability and efficacy of this compound .
生化学分析
Biochemical Properties
Phe-gly-gly plays a significant role in biochemical reactions, particularly in the context of peptide interactions and enzymatic processes. It interacts with enzymes such as peptidases, which are responsible for the hydrolysis of peptide bonds. The interaction between this compound and peptidases involves the cleavage of the peptide bond, releasing individual amino acids or smaller peptides. Additionally, this compound can interact with proteins and other biomolecules through hydrogen bonding, hydrophobic interactions, and π-π stacking interactions, which contribute to its stability and functionality in biochemical systems .
Cellular Effects
This compound influences various cellular processes, including cell signaling pathways, gene expression, and cellular metabolism. In particular, this compound has been shown to affect the proliferation and differentiation of certain cell types. For example, studies have demonstrated that this compound can modulate the activity of signaling pathways involved in cell growth and apoptosis, leading to changes in gene expression and metabolic activity . These effects highlight the potential of this compound as a modulator of cellular functions and its relevance in biomedical research.
Molecular Mechanism
The molecular mechanism of this compound involves its interactions with biomolecules at the molecular level. This compound can bind to specific receptors or enzymes, leading to the activation or inhibition of their functions. For instance, this compound can act as a competitive inhibitor of certain enzymes, preventing the binding of their natural substrates and thereby modulating their activity . Additionally, this compound can influence gene expression by interacting with transcription factors or other regulatory proteins, leading to changes in the transcriptional activity of target genes.
Temporal Effects in Laboratory Settings
In laboratory settings, the effects of this compound can vary over time due to factors such as stability and degradation. This compound is relatively stable under standard laboratory conditions, but it can undergo degradation over extended periods, particularly in the presence of proteolytic enzymes . Long-term studies have shown that this compound can have sustained effects on cellular functions, including prolonged modulation of signaling pathways and gene expression. These temporal effects are important considerations for experimental design and interpretation of results.
Dosage Effects in Animal Models
The effects of this compound in animal models are dose-dependent, with varying outcomes observed at different dosages. At low doses, this compound can enhance certain physiological processes, such as improving metabolic efficiency and promoting tissue repair . At high doses, this compound may exhibit toxic or adverse effects, including disruptions in cellular homeostasis and induction of oxidative stress . Understanding the dosage effects of this compound is crucial for its potential therapeutic applications and safety assessments.
Metabolic Pathways
This compound is involved in several metabolic pathways, including those related to amino acid metabolism and peptide degradation. It can be metabolized by enzymes such as peptidases and aminopeptidases, which cleave the peptide bonds and release free amino acids . These metabolic pathways are essential for the regulation of this compound levels in biological systems and its integration into broader metabolic networks.
Transport and Distribution
The transport and distribution of this compound within cells and tissues involve specific transporters and binding proteins. This compound can be transported across cell membranes by peptide transporters, which facilitate its uptake and distribution within cells . Once inside the cell, this compound can interact with intracellular proteins and be distributed to various cellular compartments, influencing its localization and accumulation.
Subcellular Localization
This compound exhibits specific subcellular localization patterns, which can affect its activity and function. It can be targeted to different cellular compartments, such as the cytoplasm, nucleus, or mitochondria, depending on the presence of targeting signals or post-translational modifications . The subcellular localization of this compound is important for its interactions with specific biomolecules and its role in cellular processes.
準備方法
Synthetic Routes and Reaction Conditions
The synthesis of Phe-gly-gly typically involves the stepwise coupling of amino acids using peptide synthesis techniques. One common method is solid-phase peptide synthesis (SPPS), which involves the following steps:
Attachment of the first amino acid: The carboxyl group of phenylalanine is attached to a solid resin support.
Deprotection: The amino group of phenylalanine is deprotected to allow coupling with the next amino acid.
Coupling: Glycine is coupled to phenylalanine using a coupling reagent such as dicyclohexylcarbodiimide (DCC) or N,N’-diisopropylcarbodiimide (DIC).
Deprotection and coupling: The process is repeated to couple the second glycine to the growing peptide chain.
Cleavage: The completed tripeptide is cleaved from the resin support and purified.
Industrial Production Methods
Industrial production of peptides like this compound often employs automated peptide synthesizers that use SPPS techniques. These machines can efficiently produce large quantities of peptides with high purity. Additionally, solution-phase peptide synthesis can be used for large-scale production, where the peptide is synthesized in solution rather than on a solid support.
化学反応の分析
Types of Reactions
Phe-gly-gly can undergo various chemical reactions, including:
Hydrolysis: The peptide bonds can be hydrolyzed by proteolytic enzymes or acidic or basic conditions, breaking the tripeptide into its constituent amino acids.
Oxidation: The phenylalanine residue can undergo oxidation reactions, particularly at the aromatic ring.
Substitution: The amino and carboxyl groups can participate in substitution reactions, forming derivatives of the tripeptide.
Common Reagents and Conditions
Hydrolysis: Enzymes such as pepsin or trypsin, or acidic conditions (e.g., hydrochloric acid) can be used.
Oxidation: Reagents like hydrogen peroxide or potassium permanganate can oxidize the phenylalanine residue.
Substitution: Reagents like acyl chlorides or anhydrides can be used for acylation reactions.
Major Products
Hydrolysis: Phenylalanine, glycine, and glycine.
Oxidation: Oxidized derivatives of phenylalanine.
Substitution: Acylated derivatives of this compound.
科学的研究の応用
Phe-gly-gly has various applications in scientific research, including:
Biochemistry: Studying peptide structure and function, enzyme-substrate interactions, and protein folding.
Medicine: Investigating peptide-based drug delivery systems and therapeutic peptides.
Nanotechnology: Exploring self-assembly properties of peptides for the development of nanomaterials and hydrogels
Industrial Applications: Using peptides in the development of biosensors, catalysts, and other industrial processes.
類似化合物との比較
Similar Compounds
Gly-gly-gly (Glycyl-glycyl-glycine): A tripeptide composed of three glycine residues.
Phe-phe (Phenylalanyl-phenylalanine): A dipeptide composed of two phenylalanine residues.
Gly-phe (Glycyl-phenylalanine): A dipeptide composed of glycine and phenylalanine.
Uniqueness
Phe-gly-gly is unique due to the presence of both phenylalanine and glycine residues, which provide a balance of hydrophobic and flexible properties. This combination allows this compound to participate in a variety of interactions and reactions, making it a versatile compound for research and industrial applications.
特性
IUPAC Name |
2-[[2-[[(2S)-2-amino-3-phenylpropanoyl]amino]acetyl]amino]acetic acid | |
---|---|---|
Source | PubChem | |
URL | https://pubchem.ncbi.nlm.nih.gov | |
Description | Data deposited in or computed by PubChem | |
InChI |
InChI=1S/C13H17N3O4/c14-10(6-9-4-2-1-3-5-9)13(20)16-7-11(17)15-8-12(18)19/h1-5,10H,6-8,14H2,(H,15,17)(H,16,20)(H,18,19)/t10-/m0/s1 | |
Source | PubChem | |
URL | https://pubchem.ncbi.nlm.nih.gov | |
Description | Data deposited in or computed by PubChem | |
InChI Key |
NAXPHWZXEXNDIW-JTQLQIEISA-N | |
Source | PubChem | |
URL | https://pubchem.ncbi.nlm.nih.gov | |
Description | Data deposited in or computed by PubChem | |
Canonical SMILES |
C1=CC=C(C=C1)CC(C(=O)NCC(=O)NCC(=O)O)N | |
Source | PubChem | |
URL | https://pubchem.ncbi.nlm.nih.gov | |
Description | Data deposited in or computed by PubChem | |
Isomeric SMILES |
C1=CC=C(C=C1)C[C@@H](C(=O)NCC(=O)NCC(=O)O)N | |
Source | PubChem | |
URL | https://pubchem.ncbi.nlm.nih.gov | |
Description | Data deposited in or computed by PubChem | |
Molecular Formula |
C13H17N3O4 | |
Source | PubChem | |
URL | https://pubchem.ncbi.nlm.nih.gov | |
Description | Data deposited in or computed by PubChem | |
DSSTOX Substance ID |
DTXSID00178213 | |
Record name | Phenylalanyl-glycyl-glycine | |
Source | EPA DSSTox | |
URL | https://comptox.epa.gov/dashboard/DTXSID00178213 | |
Description | DSSTox provides a high quality public chemistry resource for supporting improved predictive toxicology. | |
Molecular Weight |
279.29 g/mol | |
Source | PubChem | |
URL | https://pubchem.ncbi.nlm.nih.gov | |
Description | Data deposited in or computed by PubChem | |
CAS No. |
23576-42-3 | |
Record name | Phenylalanylglycylglycine | |
Source | CAS Common Chemistry | |
URL | https://commonchemistry.cas.org/detail?cas_rn=23576-42-3 | |
Description | CAS Common Chemistry is an open community resource for accessing chemical information. Nearly 500,000 chemical substances from CAS REGISTRY cover areas of community interest, including common and frequently regulated chemicals, and those relevant to high school and undergraduate chemistry classes. This chemical information, curated by our expert scientists, is provided in alignment with our mission as a division of the American Chemical Society. | |
Explanation | The data from CAS Common Chemistry is provided under a CC-BY-NC 4.0 license, unless otherwise stated. | |
Record name | Phenylalanyl-glycyl-glycine | |
Source | ChemIDplus | |
URL | https://pubchem.ncbi.nlm.nih.gov/substance/?source=chemidplus&sourceid=0023576423 | |
Description | ChemIDplus is a free, web search system that provides access to the structure and nomenclature authority files used for the identification of chemical substances cited in National Library of Medicine (NLM) databases, including the TOXNET system. | |
Record name | Phenylalanyl-glycyl-glycine | |
Source | EPA DSSTox | |
URL | https://comptox.epa.gov/dashboard/DTXSID00178213 | |
Description | DSSTox provides a high quality public chemistry resource for supporting improved predictive toxicology. | |
Retrosynthesis Analysis
AI-Powered Synthesis Planning: Our tool employs the Template_relevance Pistachio, Template_relevance Bkms_metabolic, Template_relevance Pistachio_ringbreaker, Template_relevance Reaxys, Template_relevance Reaxys_biocatalysis model, leveraging a vast database of chemical reactions to predict feasible synthetic routes.
One-Step Synthesis Focus: Specifically designed for one-step synthesis, it provides concise and direct routes for your target compounds, streamlining the synthesis process.
Accurate Predictions: Utilizing the extensive PISTACHIO, BKMS_METABOLIC, PISTACHIO_RINGBREAKER, REAXYS, REAXYS_BIOCATALYSIS database, our tool offers high-accuracy predictions, reflecting the latest in chemical research and data.
Strategy Settings
Precursor scoring | Relevance Heuristic |
---|---|
Min. plausibility | 0.01 |
Model | Template_relevance |
Template Set | Pistachio/Bkms_metabolic/Pistachio_ringbreaker/Reaxys/Reaxys_biocatalysis |
Top-N result to add to graph | 6 |
Feasible Synthetic Routes
試験管内研究製品の免責事項と情報
BenchChemで提示されるすべての記事および製品情報は、情報提供を目的としています。BenchChemで購入可能な製品は、生体外研究のために特別に設計されています。生体外研究は、ラテン語の "in glass" に由来し、生物体の外で行われる実験を指します。これらの製品は医薬品または薬として分類されておらず、FDAから任何の医療状態、病気、または疾患の予防、治療、または治癒のために承認されていません。これらの製品を人間または動物に体内に導入する形態は、法律により厳格に禁止されています。これらのガイドラインに従うことは、研究と実験において法的および倫理的な基準の遵守を確実にするために重要です。