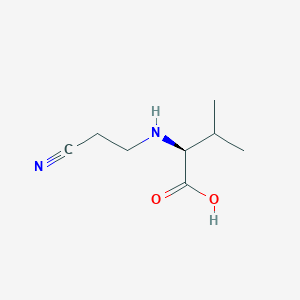
N-(2-シアノエチル)-L-バリン
概要
説明
N-(2-Cyanoethyl)-L-valine is a modified amino acid that arises from the reaction of acrylonitrile with the N-terminal valine in hemoglobin. This compound is often used as a biomarker to monitor exposure to acrylonitrile, a byproduct of cigarette smoke. It has a molecular formula of C8H14N2O2 and a molecular weight of 170.209 g/mol .
科学的研究の応用
N-(2-Cyanoethyl)-L-valine has several scientific research applications:
Chemistry: Used as a biomarker to monitor exposure to acrylonitrile.
Biology: Helps in studying the effects of acrylonitrile on biological systems.
Medicine: Used in research related to smoking and its impact on health.
Industry: Employed in the production of various chemical intermediates
作用機序
Target of Action
N-(2-Cyanoethyl)-L-valine is a derivative of natural or synthetic nucleosides . It is used in the synthesis of oligonucleotides, relatively short fragments of nucleic acid and their analogs . The primary targets of N-(2-Cyanoethyl)-L-valine are the nucleosides in the DNA or RNA strands .
Mode of Action
N-(2-Cyanoethyl)-L-valine interacts with its targets through a process known as phosphoramidite chemistry . This chemistry involves the treatment of a protected nucleoside bearing a single free hydroxy group with phosphorodiamidite under the catalytic action of a weak acid . This process allows for the sequential addition of new bases to the DNA chain in a cyclic reaction .
Biochemical Pathways
The biochemical pathway involved in the action of N-(2-Cyanoethyl)-L-valine is the synthesis of oligonucleotides . This process mimics natural processes and allows for the accurate assembly of custom oligonucleotide sequences . The 2-cyanoethyl group is employed to protect a phosphate group in the context of a synthesis .
Pharmacokinetics
The pharmacokinetics of N-(2-Cyanoethyl)-L-valine involves its rapid absorption and distribution throughout the body . After administration, it causes sustained elevations of phenylethylamine concentrations and elimination of phenylethylamine is markedly decreased in these tissues relative to the situation after administration of phenylethylamine itself .
Result of Action
The result of the action of N-(2-Cyanoethyl)-L-valine is the formation of oligonucleotides . These oligonucleotides can be used in various applications such as gene expression studies, diagnostics, and therapeutics . N-(2-Cyanoethyl)-L-valine is a result of the exposure to acrylonitrile and is used as a biomarker of long-term or historical exposure to acrylonitrile .
Action Environment
The action of N-(2-Cyanoethyl)-L-valine is influenced by environmental factors such as the presence of other chemicals and the pH of the environment . For instance, the presence of acrylonitrile can lead to the formation of N-(2-Cyanoethyl)-L-valine . Additionally, the pH of the environment can affect the rate of the phosphoramidite chemistry involved in the action of N-(2-Cyanoethyl)-L-valine .
準備方法
Synthetic Routes and Reaction Conditions: N-(2-Cyanoethyl)-L-valine can be synthesized through a two-step reaction process:
Stage 1: L-valine is reacted with potassium hydroxide in water at 20°C.
Stage 2: Acrylonitrile is added to the reaction mixture at 0-5°C and stirred for 4.5-5.5 hours.
Industrial Production Methods: The industrial production of N-(2-Cyanoethyl)-L-valine follows similar synthetic routes but on a larger scale, ensuring precise control over reaction conditions to maintain product purity and yield.
化学反応の分析
Types of Reactions: N-(2-Cyanoethyl)-L-valine undergoes several types of chemical reactions, including:
Substitution Reactions: The cyano group can be substituted with other nucleophiles.
Oxidation and Reduction Reactions: The compound can undergo oxidation and reduction under specific conditions.
Common Reagents and Conditions:
Substitution Reactions: Typically involve nucleophiles such as amines or thiols.
Oxidation Reactions: Often use oxidizing agents like hydrogen peroxide or potassium permanganate.
Reduction Reactions: Commonly employ reducing agents such as sodium borohydride or lithium aluminum hydride.
Major Products Formed: The major products formed from these reactions depend on the specific reagents and conditions used. For example, substitution reactions can yield various derivatives of N-(2-Cyanoethyl)-L-valine .
類似化合物との比較
N-(2-Hydroxyethyl)valine: Formed from the reaction of ethylene oxide with valine.
N-(2-Cyanoethyl)cysteine: Formed from the reaction of acrylonitrile with cysteine.
Uniqueness: N-(2-Cyanoethyl)-L-valine is unique due to its specific formation from acrylonitrile and valine, making it a precise biomarker for acrylonitrile exposure. Its structure and reactivity differ from other similar compounds, providing distinct analytical and research advantages .
生物活性
N-(2-Cyanoethyl)-L-valine (CEV) is a modified amino acid that primarily serves as a biomarker for exposure to acrylonitrile (ACN), a compound associated with various industrial processes and cigarette smoke. This article delves into the biological activity of CEV, focusing on its formation, detection, implications for health, and relevant research findings.
Formation and Detection
N-(2-Cyanoethyl)-L-valine is formed when acrylonitrile reacts with the N-terminal amino group of valine in hemoglobin. This reaction is significant as it provides a means to monitor exposure to acrylonitrile in humans. The detection of CEV in biological samples, particularly blood, serves as an indicator of long-term exposure to ACN.
Detection Methods
The primary method for quantifying CEV levels involves gas chromatography-mass spectrometry (GC-MS). A modified Edman degradation procedure is used to release CEV from hemoglobin for analysis. The detection limits for CEV have been established at approximately 1 pmol per gram of globin, with quantification limits around 60 pmol/g .
Mechanism of Toxicity
The biological activity of CEV is closely linked to the toxic effects of acrylonitrile. Once in the body, acrylonitrile can be metabolized into cyanide, which disrupts cellular respiration by inhibiting cytochrome c oxidase in the mitochondrial electron transport chain. This inhibition leads to decreased ATP production, particularly affecting tissues reliant on aerobic metabolism, such as the central nervous system and heart .
Health Implications
Research has shown that elevated levels of CEV are associated with various health risks:
- Carcinogenic Potential : Studies indicate that chronic exposure to acrylonitrile correlates with an increased incidence of cancers in laboratory animals. For instance, long-term studies in rats have demonstrated significant tumor development at doses ranging from 0.1 to 2 mg/kg body weight .
- Neurological Effects : High levels of CEV have been linked to peripheral neurotoxic symptoms in exposed populations. A study involving workers exposed to both acrylonitrile and acrylamide found a correlation between CEV levels and neurological symptoms, highlighting the potential neurotoxic effects of these compounds .
Case Studies
Several case studies illustrate the biological activity and implications of N-(2-Cyanoethyl)-L-valine:
- Occupational Exposure : In a cohort study involving workers exposed to acrylonitrile, average CEV levels were significantly higher than those in control groups. The study reported mean values of 1984 ± 2066 pmol/g globin for exposed workers compared to 31.1 ± 18.5 pmol/g globin in controls .
- Maternal-Fetal Transfer : Research examining pregnant smokers revealed that maternal smoking correlated with elevated CEV levels in both mothers and their newborns. This transplacental transfer indicates potential developmental risks associated with maternal exposure to acrylonitrile .
Research Findings Summary
The following table summarizes key findings related to N-(2-Cyanoethyl)-L-valine:
特性
IUPAC Name |
(2S)-2-(2-cyanoethylamino)-3-methylbutanoic acid | |
---|---|---|
Source | PubChem | |
URL | https://pubchem.ncbi.nlm.nih.gov | |
Description | Data deposited in or computed by PubChem | |
InChI |
InChI=1S/C8H14N2O2/c1-6(2)7(8(11)12)10-5-3-4-9/h6-7,10H,3,5H2,1-2H3,(H,11,12)/t7-/m0/s1 | |
Source | PubChem | |
URL | https://pubchem.ncbi.nlm.nih.gov | |
Description | Data deposited in or computed by PubChem | |
InChI Key |
RXNXODTWPQTIHO-ZETCQYMHSA-N | |
Source | PubChem | |
URL | https://pubchem.ncbi.nlm.nih.gov | |
Description | Data deposited in or computed by PubChem | |
Canonical SMILES |
CC(C)C(C(=O)O)NCCC#N | |
Source | PubChem | |
URL | https://pubchem.ncbi.nlm.nih.gov | |
Description | Data deposited in or computed by PubChem | |
Isomeric SMILES |
CC(C)[C@@H](C(=O)O)NCCC#N | |
Source | PubChem | |
URL | https://pubchem.ncbi.nlm.nih.gov | |
Description | Data deposited in or computed by PubChem | |
Molecular Formula |
C8H14N2O2 | |
Source | PubChem | |
URL | https://pubchem.ncbi.nlm.nih.gov | |
Description | Data deposited in or computed by PubChem | |
DSSTOX Substance ID |
DTXSID90470238 | |
Record name | N-(2-Cyanoethyl)valine | |
Source | EPA DSSTox | |
URL | https://comptox.epa.gov/dashboard/DTXSID90470238 | |
Description | DSSTox provides a high quality public chemistry resource for supporting improved predictive toxicology. | |
Molecular Weight |
170.21 g/mol | |
Source | PubChem | |
URL | https://pubchem.ncbi.nlm.nih.gov | |
Description | Data deposited in or computed by PubChem | |
CAS No. |
51078-49-0 | |
Record name | N-(2-Cyanoethyl)valine | |
Source | EPA DSSTox | |
URL | https://comptox.epa.gov/dashboard/DTXSID90470238 | |
Description | DSSTox provides a high quality public chemistry resource for supporting improved predictive toxicology. | |
Retrosynthesis Analysis
AI-Powered Synthesis Planning: Our tool employs the Template_relevance Pistachio, Template_relevance Bkms_metabolic, Template_relevance Pistachio_ringbreaker, Template_relevance Reaxys, Template_relevance Reaxys_biocatalysis model, leveraging a vast database of chemical reactions to predict feasible synthetic routes.
One-Step Synthesis Focus: Specifically designed for one-step synthesis, it provides concise and direct routes for your target compounds, streamlining the synthesis process.
Accurate Predictions: Utilizing the extensive PISTACHIO, BKMS_METABOLIC, PISTACHIO_RINGBREAKER, REAXYS, REAXYS_BIOCATALYSIS database, our tool offers high-accuracy predictions, reflecting the latest in chemical research and data.
Strategy Settings
Precursor scoring | Relevance Heuristic |
---|---|
Min. plausibility | 0.01 |
Model | Template_relevance |
Template Set | Pistachio/Bkms_metabolic/Pistachio_ringbreaker/Reaxys/Reaxys_biocatalysis |
Top-N result to add to graph | 6 |
Feasible Synthetic Routes
試験管内研究製品の免責事項と情報
BenchChemで提示されるすべての記事および製品情報は、情報提供を目的としています。BenchChemで購入可能な製品は、生体外研究のために特別に設計されています。生体外研究は、ラテン語の "in glass" に由来し、生物体の外で行われる実験を指します。これらの製品は医薬品または薬として分類されておらず、FDAから任何の医療状態、病気、または疾患の予防、治療、または治癒のために承認されていません。これらの製品を人間または動物に体内に導入する形態は、法律により厳格に禁止されています。これらのガイドラインに従うことは、研究と実験において法的および倫理的な基準の遵守を確実にするために重要です。