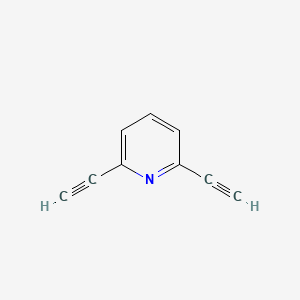
2,6-Diethynylpyridine
説明
2,6-Diethynylpyridine is a chemical compound with the molecular formula C9H5N . It has a molecular weight of 127.14 g/mol . The compound is characterized by the absence of alkyl chains and conventional hydrogen bonding sites .
Synthesis Analysis
The synthesis of 2,6-Diethynylpyridine involves a cascade reaction between N-tosylhydrazones and 2-alkynylpyridines . When the reaction is conducted with 2,6-diethynylpyridine, 2,6-bis(pyrazolyl)pyridines are obtained . Another method involves the use of 2,6-diethynylpyridine and 2-azidoethylphosphonic acid .
Molecular Structure Analysis
The molecular structure of 2,6-Diethynylpyridine is characterized by a 2D structure and a 3D conformer . The compound forms an ordered array at the interface between 1-phenyloctane and highly oriented pyrolytic graphite (HOPG) under room temperature conditions .
Physical And Chemical Properties Analysis
2,6-Diethynylpyridine has a density of 1.1±0.1 g/cm³, a boiling point of 233.9±25.0 °C at 760 mmHg, and a vapor pressure of 0.1±0.4 mmHg at 25°C . It has a molar refractivity of 38.7±0.4 cm³ and a polar surface area of 13 Ų . The compound also has a molar volume of 118.0±5.0 cm³ .
科学的研究の応用
Here is a comprehensive analysis of the scientific research applications of 2,6-Diethynylpyridine:
High Pressure Polymerization
2,6-Diethynylpyridine has been used in high-pressure polymerization to produce novel carbon materials such as diamond nanowires and graphene. The introduction of conjugated alkynyl groups reduces the reaction pressure required for polymerization of aromatic molecules, allowing for the formation of one-dimensional ordered polymers at pressures below 10 GPa .
Polar Assembly and Crystallization
This compound crystallizes to form C(sp²)–H···N hydrogen-bonded head-to-tail tapes, which assemble into two-dimensional polar sheets through C(sp)–H···π hydrogen bridges. Further assembly through π–π stacking interactions results in three-dimensional polar crystals with a strong powder second harmonic generation (SHG) response .
Porous Organic Frameworks
2,6-Diethynylpyridine reacts with tetrakis(4-azidophenyl)methane to create a porous organic framework containing chelating terdentate 2,6-bis(1,2,3-triazol-4-yl)pyridyl units. This framework can serve as a promising linkage for organic frameworks and as an effective stabilizer for palladium nanoparticles .
Safety and Hazards
If inhaled, the victim should be moved into fresh air. If breathing is difficult, oxygen should be given. If not breathing, artificial respiration should be given and a doctor should be consulted immediately . Following skin contact, contaminated clothing should be removed immediately and the skin should be washed off with soap and plenty of water . Following eye contact, the eyes should be rinsed with pure water for at least 15 minutes .
特性
IUPAC Name |
2,6-diethynylpyridine | |
---|---|---|
Source | PubChem | |
URL | https://pubchem.ncbi.nlm.nih.gov | |
Description | Data deposited in or computed by PubChem | |
InChI |
InChI=1S/C9H5N/c1-3-8-6-5-7-9(4-2)10-8/h1-2,5-7H | |
Source | PubChem | |
URL | https://pubchem.ncbi.nlm.nih.gov | |
Description | Data deposited in or computed by PubChem | |
InChI Key |
VYRLFYTZNXGQIK-UHFFFAOYSA-N | |
Source | PubChem | |
URL | https://pubchem.ncbi.nlm.nih.gov | |
Description | Data deposited in or computed by PubChem | |
Canonical SMILES |
C#CC1=NC(=CC=C1)C#C | |
Source | PubChem | |
URL | https://pubchem.ncbi.nlm.nih.gov | |
Description | Data deposited in or computed by PubChem | |
Molecular Formula |
C9H5N | |
Source | PubChem | |
URL | https://pubchem.ncbi.nlm.nih.gov | |
Description | Data deposited in or computed by PubChem | |
DSSTOX Substance ID |
DTXSID30503251 | |
Record name | 2,6-Diethynylpyridine | |
Source | EPA DSSTox | |
URL | https://comptox.epa.gov/dashboard/DTXSID30503251 | |
Description | DSSTox provides a high quality public chemistry resource for supporting improved predictive toxicology. | |
Molecular Weight |
127.14 g/mol | |
Source | PubChem | |
URL | https://pubchem.ncbi.nlm.nih.gov | |
Description | Data deposited in or computed by PubChem | |
Product Name |
2,6-Diethynylpyridine | |
CAS RN |
75867-46-8 | |
Record name | 2,6-Diethynylpyridine | |
Source | EPA DSSTox | |
URL | https://comptox.epa.gov/dashboard/DTXSID30503251 | |
Description | DSSTox provides a high quality public chemistry resource for supporting improved predictive toxicology. | |
Record name | 2,6-Diethynylpyridine | |
Source | European Chemicals Agency (ECHA) | |
URL | https://echa.europa.eu/information-on-chemicals | |
Description | The European Chemicals Agency (ECHA) is an agency of the European Union which is the driving force among regulatory authorities in implementing the EU's groundbreaking chemicals legislation for the benefit of human health and the environment as well as for innovation and competitiveness. | |
Explanation | Use of the information, documents and data from the ECHA website is subject to the terms and conditions of this Legal Notice, and subject to other binding limitations provided for under applicable law, the information, documents and data made available on the ECHA website may be reproduced, distributed and/or used, totally or in part, for non-commercial purposes provided that ECHA is acknowledged as the source: "Source: European Chemicals Agency, http://echa.europa.eu/". Such acknowledgement must be included in each copy of the material. ECHA permits and encourages organisations and individuals to create links to the ECHA website under the following cumulative conditions: Links can only be made to webpages that provide a link to the Legal Notice page. | |
Retrosynthesis Analysis
AI-Powered Synthesis Planning: Our tool employs the Template_relevance Pistachio, Template_relevance Bkms_metabolic, Template_relevance Pistachio_ringbreaker, Template_relevance Reaxys, Template_relevance Reaxys_biocatalysis model, leveraging a vast database of chemical reactions to predict feasible synthetic routes.
One-Step Synthesis Focus: Specifically designed for one-step synthesis, it provides concise and direct routes for your target compounds, streamlining the synthesis process.
Accurate Predictions: Utilizing the extensive PISTACHIO, BKMS_METABOLIC, PISTACHIO_RINGBREAKER, REAXYS, REAXYS_BIOCATALYSIS database, our tool offers high-accuracy predictions, reflecting the latest in chemical research and data.
Strategy Settings
Precursor scoring | Relevance Heuristic |
---|---|
Min. plausibility | 0.01 |
Model | Template_relevance |
Template Set | Pistachio/Bkms_metabolic/Pistachio_ringbreaker/Reaxys/Reaxys_biocatalysis |
Top-N result to add to graph | 6 |
Feasible Synthetic Routes
Q & A
ANone: 2,6-diethynylpyridine (C₉H₅N) has a molecular weight of 127.14 g/mol. It is characterized by a pyridine ring with two ethynyl groups attached at the 2 and 6 positions. Spectroscopic data, including NMR and UV-Vis, can be found in various publications [].
ANone: While lacking conventional hydrogen bonding sites and alkyl chains, 2,6-diethynylpyridine exhibits self-assembly into ordered structures at specific interfaces. For instance, at the 1-phenyloctane/highly oriented pyrolytic graphite (HOPG) interface, it forms ordered arrays driven by weak C-H...N and C-H...π hydrogen bonds, influenced by the periodic potential of the graphite surface []. This molecule also exhibits polar assembly in its crystal structure through a combination of C(sp2)-H...N hydrogen bonds, C(sp)-H...π hydrogen bridges, and π-π stacking interactions, leading to a 3D polar crystal [].
ANone: The polar assembly of 2,6-diethynylpyridine crystals results in notable nonlinear optical properties. Specifically, it exhibits a strong powder second harmonic generation (SHG) response, found to be five times stronger than that of crystalline urea [], highlighting its potential in nonlinear optics applications.
ANone: Yes, 2,6-diethynylpyridine serves as a valuable building block in polymer synthesis. It is widely employed in Sonogashira-Hagihara coupling reactions, enabling the creation of diverse polymers. For instance, its reaction with cis-3,6-diethynyl-3,6-dimethoxycyclohexa-1,4-diene or cis-9,10-diethynyl-9,10-dimethoxy-9,10-dihydroanthracene yields macrocyclic compounds with alternating segments []. Additionally, it has been successfully incorporated into the synthesis of poly(N-isopropylacrylamide) (PNIPAM) to create both covalently linked and non-covalently Ru(II)-chelated four-arm star-shaped polymers [, ].
ANone: Quaternization of the pyridine nitrogen in 2,6-diethynylpyridine, achieved through reactions with methyl iodide or methyl triflate, significantly alters its electronic and optical properties. This modification leads to enhanced π-electron delocalization along the molecule's backbone, resulting in a pronounced red shift in UV-Vis absorption spectra [, ]. Additionally, quaternized derivatives often display increased fluorescence intensities and quantum efficiencies compared to their neutral counterparts [].
ANone: 2,6-diethynylpyridine acts as a key component in constructing metallo-supramolecular structures. Its ability to chelate with metal ions, like Ni2+, through the nitrogen atom of the pyridine ring and the triple bonds of the ethynyl groups enables the formation of supramolecular hydrogels []. The self-assembly process is driven by the coordination interactions between the ligand and the metal ions, leading to the formation of three-dimensional networks that trap water molecules, resulting in hydrogel formation.
ANone: Research indicates promising catalytic applications for materials incorporating 2,6-diethynylpyridine. For example, a porous organic framework (POF) synthesized using 2,6-diethynylpyridine as a building block has demonstrated excellent catalytic activity in the palladium-catalyzed hydrogenation of olefins []. The 2,6-bis(1,2,3-triazol-4-yl)pyridyl units within the POF act as chelating sites for palladium nanoparticles, facilitating their stabilization and dispersion within the porous framework.
ANone: The geometry of 2,6-diethynylpyridine, along with the counter anion used, plays a crucial role in determining the dimensionality of the resulting organometallic networks when complexed with silver(I) []. For instance, reactions with 2,5-diethynylpyridine (a positional isomer) can yield either 2D or 3D networks based on the anion employed (CF3CO2- vs. NO3-). In contrast, 2,6-diethynylpyridine, with its ethynyl groups at the 2 and 6 positions, leads to the formation of organometallic chains when reacted with CF3CO2Ag. These chains are further interconnected through the CF3CO2- ligands, ultimately resulting in a 2D network [].
ANone: Derivatives of 2,6-diethynylpyridine, specifically bis(phenylethynyl)pyridylcarboxamides, have shown potential as environment-sensitive fluorescent probes for biological applications []. These probes exhibit significant fluorescence turn-on responses upon binding to specific DNA structures, such as the human telomeric G-quadruplex (h-TELO) []. This property enables their use in detecting and imaging these biologically relevant DNA structures with high selectivity.
ANone: Click chemistry, particularly the copper(I)-catalyzed alkyne-azide cycloaddition (CuAAC) reaction, has proven highly advantageous in synthesizing various 2,6-diethynylpyridine-based materials [, , ]. This approach offers several benefits:
ANone: Supramolecular hydrogels based on 2,6-diethynylpyridine showcase a remarkable ability to self-heal, attributed to the dynamic nature of the metal-ligand coordination bonds []. When a hydrogel incorporating this ligand is damaged, the non-covalent interactions between the 2,6-bis(1,2,3-triazol-4-yl)-pyridine (btp) ligand and metal ions, such as Ni2+, can readily break and reform at the damaged site, enabling the hydrogel to regain its structural integrity and mechanical properties over time []. This self-healing capacity holds potential for applications in areas like drug delivery, wound healing, and tissue engineering.
試験管内研究製品の免責事項と情報
BenchChemで提示されるすべての記事および製品情報は、情報提供を目的としています。BenchChemで購入可能な製品は、生体外研究のために特別に設計されています。生体外研究は、ラテン語の "in glass" に由来し、生物体の外で行われる実験を指します。これらの製品は医薬品または薬として分類されておらず、FDAから任何の医療状態、病気、または疾患の予防、治療、または治癒のために承認されていません。これらの製品を人間または動物に体内に導入する形態は、法律により厳格に禁止されています。これらのガイドラインに従うことは、研究と実験において法的および倫理的な基準の遵守を確実にするために重要です。