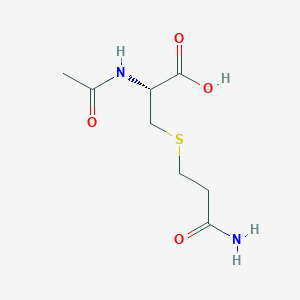
N-アセチル-S-(2-カルバモイルエチル)-L-システイン
概要
説明
N-Acetyl-S-(2-carbamoylethyl)-cysteine is a N-acyl-L-amino acid.
科学的研究の応用
アクリルアミド曝露のバイオマーカー
AAMAは、人体におけるアクリルアミド(AA)の主要な代謝産物です . そのため、それはしばしばヒトのAA曝露を評価するための生物学的指標として使用されます . これは特に重要です。なぜなら、AAは高温で加工された食品に存在する、ヒトに対する発がん性がある可能性のある物質であり、世界中でヒトにおいて頻繁に検出されています .
尿サンプルにおける検出
AAMAは、高速液体クロマトグラフィーを使用して尿サンプルで検出できます . 酸加水分解後、尿中のAAMAはS-2-カルボキシエチルシステインを形成し、これはo-フタラールデヒド誘導体試薬と反応して、強い蛍光吸光体を形成します . この方法は、シンプルで、費用対効果が高く、感度も高いことから、幅広い用途に適しています .
食品消費との関連性
研究によると、クラッカーやチョコレートなどの特定の食品の消費は、尿中のAAMA濃度と有意に関連しています . これは、食習慣が身体のAA曝露に影響を与える可能性があることを示唆しています
作用機序
Target of Action
N-Acetyl-S-(2-carbamoylethyl)-L-cysteine (AAMA) is a major metabolite of acrylamide (AA), a probable human carcinogen . The primary targets of AAMA are proteins, plasma albumin, and valine amino acids of hemoglobin .
Mode of Action
AAMA is formed as a result of the metabolic conversion of acrylamide in the body . Acrylamide and its metabolite glycidamide are identified as alkylating agents which can easily react with proteins, plasma albumin, and valine amino acids of hemoglobin to form N-(2-carbamoyl-2-hydroxyethyl) valine (HbGA) and N-(2-carbamoylethyl) valine (HbAA) .
Biochemical Pathways
The metabolic formation of glycidamide from acrylamide is considered as activating biotransformation responsible for genotoxicity, mutagenicity, and carcinogenicity of the parent molecule, acrylamide . The N7-Gua adduct, N7-(2 carbamoyl-2-hydroxy ethyl) guanine (N7-GA-Gua) is by far the most abundant DNA adduct derived from glycidamide by covalent interaction .
Pharmacokinetics
The pharmacokinetics of AAMA involves its formation through the metabolic conversion of acrylamide. After acid hydrolysis, AAMA in urine is converted into S-2-carboxyethyl cysteine . The concentrations of AAMA in urine can range between 15.4 and 196.3 ng/mL, and the levels can vary by day considerably even in a given individual .
Result of Action
The molecular and cellular effects of AAMA’s action are primarily related to its genotoxic and mutagenic properties. It forms adducts with DNA and proteins, which can lead to mutations and potentially contribute to carcinogenesis .
Action Environment
Environmental factors such as diet and exposure to smoke can influence the action, efficacy, and stability of AAMA. For instance, children exposed to environmental smoke at home exhibited significantly higher levels of AAMA in urine, suggesting the importance of passive smoking as a source of acrylamide exposure . Additionally, certain foods, such as crackers and chocolate, have been identified to be significantly associated with the concentrations of AAMA in urine .
Safety and Hazards
Acrylamide, from which AAMA is derived, is widely found in a variety of fried foods and cigarettes. It is not only neurotoxic and carcinogenic, but also has many potential toxic effects . The current assessment of acrylamide intake through dietary questionnaires is confounded by a variety of factors, which poses limitations to safety assessment .
将来の方向性
AAMA, as a biomarker of internal human exposure, can reflect acrylamide intake in the short term. This is of great significance for tracing acrylamide-containing foods and setting the allowable intake of acrylamide in foods . Therefore, future research could focus on the levels of AAMA in humans and its association with other diseases .
生化学分析
Biochemical Properties
N-Acetyl-S-(2-carbamoylethyl)-L-cysteine interacts with various enzymes and proteins in the body. It is a product of the metabolism of acrylamide, which involves the action of cytochrome P450 enzymes . The formation of N-Acetyl-S-(2-carbamoylethyl)-L-cysteine is an important step in the detoxification of acrylamide .
Cellular Effects
The presence of N-Acetyl-S-(2-carbamoylethyl)-L-cysteine in cells is indicative of acrylamide exposure. Acrylamide has been shown to have neurotoxic effects, causing peripheral neuropathy, and it is also considered a potential carcinogen . The levels of N-Acetyl-S-(2-carbamoylethyl)-L-cysteine can therefore have implications for cell function and health.
Molecular Mechanism
N-Acetyl-S-(2-carbamoylethyl)-L-cysteine is formed through the action of cytochrome P450 enzymes on acrylamide, specifically through the action of the CYP2E1 enzyme . This transformation is part of the body’s mechanism for detoxifying acrylamide.
Temporal Effects in Laboratory Settings
The levels of N-Acetyl-S-(2-carbamoylethyl)-L-cysteine in urine can vary significantly, even within a single individual . This suggests that the metabolism of acrylamide and the formation of N-Acetyl-S-(2-carbamoylethyl)-L-cysteine can be influenced by various factors, including the individual’s diet and exposure to acrylamide .
Dosage Effects in Animal Models
The effects of N-Acetyl-S-(2-carbamoylethyl)-L-cysteine in animal models are largely reflective of acrylamide exposure. High levels of acrylamide can lead to neurotoxic effects and potential carcinogenicity . Therefore, high levels of N-Acetyl-S-(2-carbamoylethyl)-L-cysteine would indicate high exposure to acrylamide.
Metabolic Pathways
N-Acetyl-S-(2-carbamoylethyl)-L-cysteine is part of the metabolic pathway for acrylamide. Acrylamide is metabolized by cytochrome P450 enzymes, specifically CYP2E1, to form glycidamide, which can then be further metabolized to form N-Acetyl-S-(2-carbamoylethyl)-L-cysteine .
Transport and Distribution
N-Acetyl-S-(2-carbamoylethyl)-L-cysteine is primarily found in urine, indicating that it is excreted from the body through the kidneys . This suggests that it is distributed through the bloodstream to the kidneys, where it is filtered out and excreted.
特性
IUPAC Name |
(2R)-2-acetamido-3-(3-amino-3-oxopropyl)sulfanylpropanoic acid | |
---|---|---|
Source | PubChem | |
URL | https://pubchem.ncbi.nlm.nih.gov | |
Description | Data deposited in or computed by PubChem | |
InChI |
InChI=1S/C8H14N2O4S/c1-5(11)10-6(8(13)14)4-15-3-2-7(9)12/h6H,2-4H2,1H3,(H2,9,12)(H,10,11)(H,13,14)/t6-/m0/s1 | |
Source | PubChem | |
URL | https://pubchem.ncbi.nlm.nih.gov | |
Description | Data deposited in or computed by PubChem | |
InChI Key |
GGBCHNJZQQEQRX-LURJTMIESA-N | |
Source | PubChem | |
URL | https://pubchem.ncbi.nlm.nih.gov | |
Description | Data deposited in or computed by PubChem | |
Canonical SMILES |
CC(=O)NC(CSCCC(=O)N)C(=O)O | |
Source | PubChem | |
URL | https://pubchem.ncbi.nlm.nih.gov | |
Description | Data deposited in or computed by PubChem | |
Isomeric SMILES |
CC(=O)N[C@@H](CSCCC(=O)N)C(=O)O | |
Source | PubChem | |
URL | https://pubchem.ncbi.nlm.nih.gov | |
Description | Data deposited in or computed by PubChem | |
Molecular Formula |
C8H14N2O4S | |
Source | PubChem | |
URL | https://pubchem.ncbi.nlm.nih.gov | |
Description | Data deposited in or computed by PubChem | |
DSSTOX Substance ID |
DTXSID30920489 | |
Record name | N-Acetyl-S-(3-amino-3-oxopropyl)cysteine | |
Source | EPA DSSTox | |
URL | https://comptox.epa.gov/dashboard/DTXSID30920489 | |
Description | DSSTox provides a high quality public chemistry resource for supporting improved predictive toxicology. | |
Molecular Weight |
234.28 g/mol | |
Source | PubChem | |
URL | https://pubchem.ncbi.nlm.nih.gov | |
Description | Data deposited in or computed by PubChem | |
CAS No. |
81690-92-8 | |
Record name | N-Acetyl-S-(propionamide)cysteine | |
Source | ChemIDplus | |
URL | https://pubchem.ncbi.nlm.nih.gov/substance/?source=chemidplus&sourceid=0081690928 | |
Description | ChemIDplus is a free, web search system that provides access to the structure and nomenclature authority files used for the identification of chemical substances cited in National Library of Medicine (NLM) databases, including the TOXNET system. | |
Record name | N-Acetyl-S-(3-amino-3-oxopropyl)cysteine | |
Source | EPA DSSTox | |
URL | https://comptox.epa.gov/dashboard/DTXSID30920489 | |
Description | DSSTox provides a high quality public chemistry resource for supporting improved predictive toxicology. | |
Retrosynthesis Analysis
AI-Powered Synthesis Planning: Our tool employs the Template_relevance Pistachio, Template_relevance Bkms_metabolic, Template_relevance Pistachio_ringbreaker, Template_relevance Reaxys, Template_relevance Reaxys_biocatalysis model, leveraging a vast database of chemical reactions to predict feasible synthetic routes.
One-Step Synthesis Focus: Specifically designed for one-step synthesis, it provides concise and direct routes for your target compounds, streamlining the synthesis process.
Accurate Predictions: Utilizing the extensive PISTACHIO, BKMS_METABOLIC, PISTACHIO_RINGBREAKER, REAXYS, REAXYS_BIOCATALYSIS database, our tool offers high-accuracy predictions, reflecting the latest in chemical research and data.
Strategy Settings
Precursor scoring | Relevance Heuristic |
---|---|
Min. plausibility | 0.01 |
Model | Template_relevance |
Template Set | Pistachio/Bkms_metabolic/Pistachio_ringbreaker/Reaxys/Reaxys_biocatalysis |
Top-N result to add to graph | 6 |
Feasible Synthetic Routes
試験管内研究製品の免責事項と情報
BenchChemで提示されるすべての記事および製品情報は、情報提供を目的としています。BenchChemで購入可能な製品は、生体外研究のために特別に設計されています。生体外研究は、ラテン語の "in glass" に由来し、生物体の外で行われる実験を指します。これらの製品は医薬品または薬として分類されておらず、FDAから任何の医療状態、病気、または疾患の予防、治療、または治癒のために承認されていません。これらの製品を人間または動物に体内に導入する形態は、法律により厳格に禁止されています。これらのガイドラインに従うことは、研究と実験において法的および倫理的な基準の遵守を確実にするために重要です。