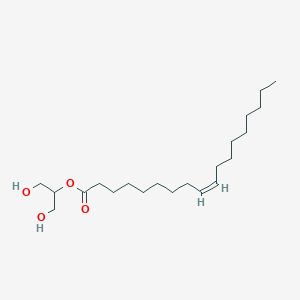
2-Monoolein
概要
説明
準備方法
合成経路と反応条件: 2-オレオイルグリセロールは、ジアシルグリセロールの酵素的加水分解によって合成できます。 反応は通常、リパーゼの使用を含み、リパーゼはジアシルグリセロールのエステル結合を選択的に加水分解してモノアシルグリセロールを生成します .
工業生産方法: 2-オレオイルグリセロールの工業生産には、通常、高純度のオレイン酸とグリセロールが使用されます。反応は、高い収率と純度を確保するために、制御された条件下でリパーゼによって触媒されます。 プロセスには、最終製品を得るために蒸留や結晶化などの精製ステップが含まれる場合もあります .
化学反応の分析
Enzymatic Ethanolysis of Oleic Acid-Rich Oils
A scalable method for synthesizing 2-monoolein involves ethanolysis of oleic acid-rich oils using lipases. Key parameters include:
Parameter | Optimal Condition | Outcome |
---|---|---|
Solvent | Hexane | Minimizes acyl migration |
Lipase Loading | 10% (w/w) | Maximizes 2-MAGs yield (37.6%) |
Reaction Time | 24 hours | Achieves equilibrium conversion |
Post-Processing | Crystallization in hexane | Purity: 97.5% Yield: 78.3% (w/w) |
This method avoids toxic solvents and achieves high regioselectivity, making it industrially viable .
Lipase-Catalyzed Hydrolysis of Triolein
Using Thermomyces lanuginosa lipase, triolein undergoes selective hydrolysis in a hexane/water system:
-
Conditions : 310 K, 24 hours, 160 rpm.
-
Yield : 0.58 g of this compound from 1.9 g triolein .
Pseudomonas fluorescens lipase favors 1,2-diolein synthesis (58% yield), highlighting enzyme specificity .
Acyl Migration
This compound undergoes acyl migration to 1-monoolein under specific conditions:
-
Solvent Polarity : Polar solvents (e.g., ethanol) accelerate migration, reducing regiochemical purity .
-
Temperature : Elevated temperatures (>40°C) increase isomerization rates .
Hydrolysis and Esterification
-
Hydrolysis : In aqueous environments, lipases cleave the ester bond, yielding oleic acid and glycerol.
-
Esterification : Reacts with fatty acids or alcohols to form diacylglycerols or structured lipids, though this is less common due to steric hindrance at the sn-2 position .
Antibacterial Activity
This compound exhibits weak antibacterial effects:
Organism | Activity Level |
---|---|
Staphylococcus aureus | Moderate |
Escherichia coli | None |
This contrasts with 1-monolinolein, which shows stronger activity .
Lipid Droplet Modulation
Monoolein-based cubosomes induce lipid droplet accumulation in cells:
-
Mechanism : Internalized monoolein is metabolized into neutral lipids.
-
Outcome : 25–31-fold increase in lipid droplet density after 24-hour exposure .
Solvent Effects on Reactivity
Solvent | Effect on this compound |
---|---|
Hexane | Stabilizes sn-2 configuration |
Ethanol | Promotes acyl migration |
Water | Facilitates hydrolysis |
Thermal Stability
Emulsification
This compound serves as a non-ionic surfactant in:
Signal Modulation
Acts as an endogenous ligand for GPR119, enhancing incretin secretion (GLP-1, GIP) .
科学的研究の応用
Food Industry
Emulsifier and Surfactant
- 2-Monoolein serves as an effective emulsifier in food products, enhancing texture and stability. Its amphiphilic nature allows it to reduce surface tension between oil and water phases, improving the consistency of emulsions .
- It is commonly used in margarine, dressings, and other emulsified products.
Pharmaceutical Applications
Drug Delivery Systems
- This compound is utilized in the development of nanocarriers for drug delivery. Its biocompatibility and ability to form liquid crystalline structures make it ideal for encapsulating hydrophobic drugs, enhancing their solubility and bioavailability .
- A notable study highlighted its application in chronic lung disease treatments, where monoolein-based nanoparticles improved drug targeting to affected tissues .
Antioxidant Properties
- Research indicates that this compound exhibits strong antioxidant effects, which can be beneficial in preventing oxidative stress-related diseases such as cardiovascular diseases .
Nanotechnology
Cubosome Formulations
- Monoolein-based cubosomes are being explored for their potential in drug delivery systems. These nanoparticles can encapsulate therapeutic agents while providing controlled release properties .
- Studies have shown that cubosome formulations stabilized with Pluronic F108 and F127 can significantly alter cellular lipid profiles and induce lipid droplet accumulation in HeLa cells, indicating their potential impact on cellular metabolism .
Case Study 1: Enzymatic Synthesis of this compound
A study detailed a scalable enzymatic method for synthesizing this compound from oleic acid-rich oils. The process yielded high purity levels while demonstrating economic advantages over traditional methods. The synthesized product was tested for its emulsifying properties in food applications, confirming its effectiveness as a surfactant .
Case Study 2: Monoolein in Drug Delivery
In a clinical investigation focusing on chronic lung diseases, monoolein was incorporated into nanoparticle formulations aimed at improving drug delivery efficacy. Results indicated enhanced therapeutic outcomes with reduced side effects compared to conventional delivery methods. The study emphasized monoolein's role in achieving targeted drug delivery to lung tissues .
Data Tables
作用機序
2-オレオイルグリセロールは、主にGタンパク質共役受容体であるGPR119の活性化によってその効果を発揮します。GPR119に結合すると、2-オレオイルグリセロールは腸のL細胞からのグルカゴン様ペプチド-1(GLP-1)の放出を刺激します。 これにより、インスリン分泌の増加とグルコース恒常性の改善につながります . さらに、2-オレオイルグリセロールは、5-HT2A受容体のアロステリック結合によるGタンパク質シグナル伝達を促進することが示されています .
類似の化合物:
2-アラキドノイルグリセロール: カンナビノイド受容体のエンドジェナスリガンドとして作用する別のモノアシルグリセロール。
1,3-ジオレオイルグリセロール: 脂質代謝の研究に使用される、2つのオレオイル基を持つジアシルグリセロール。
2-パルミトイルグリセロール: さまざまな代謝経路に関与する、パルミトイル基を持つモノアシルグリセロール
2-オレオイルグリセロールの独自性: 2-オレオイルグリセロールは、他のモノアシルグリセロールとは異なるGPR119との特異的な相互作用によりユニークです。 GLP-1の放出を刺激し、グルコース代謝を調節する能力により、代謝性疾患の治療への応用の有望な候補となっています .
類似化合物との比較
2-Arachidonoyl glycerol: Another monoacylglycerol that acts as an endogenous ligand for cannabinoid receptors.
1,3-Dioleoyl glycerol: A diacylglycerol with two oleoyl groups, used in studies of lipid metabolism.
2-Palmitoyl glycerol: A monoacylglycerol with a palmitoyl group, involved in various metabolic pathways
Uniqueness of 2-Oleoyl Glycerol: 2-Oleoyl glycerol is unique due to its specific interaction with GPR119, which distinguishes it from other monoacylglycerols. Its ability to stimulate GLP-1 release and regulate glucose metabolism makes it a promising candidate for therapeutic applications in metabolic disorders .
生物活性
2-Monoolein, a monoacylglycerol derived from oleic acid, has garnered significant attention due to its diverse biological activities and potential applications in various fields such as pharmaceuticals, food science, and cosmetics. This article explores the biological activity of this compound, highlighting its mechanisms of action, therapeutic potentials, and relevant research findings.
This compound (CAS 3443-84-3) is characterized by its structure as a glycerol molecule esterified with oleic acid at the sn-2 position. Its synthesis can be achieved through enzymatic methods that yield high purity and efficiency, making it suitable for industrial applications. Recent studies report that the enzymatic production of this compound can achieve purities of up to 98% with significant yields, facilitating its use as an emulsifier and surfactant in various formulations .
Mechanisms of Biological Activity
The biological activity of this compound can be attributed to several mechanisms:
- Cellular Uptake and Lipid Metabolism : Research indicates that this compound influences cellular lipid profiles significantly. For instance, studies involving HeLa cells demonstrated that treatment with monoolein-based cubosome formulations led to increased lipid droplet accumulation and modifications in mitochondrial function, including hyperpolarization and reactive oxygen species (ROS) generation .
- Antioxidant Properties : this compound exhibits strong antioxidant activity, which may contribute to its potential in treating oxidative stress-related conditions. In vitro studies have shown its efficacy in reducing oxidative damage in cellular models .
- Anti-Atherogenic Effects : The compound has also been noted for its anti-atherosclerotic properties, suggesting potential applications in cardiovascular health management .
Case Studies
-
Cellular Response to Monoolein-Based Formulations :
- A study investigated the effects of monoolein cubosomes on HeLa cells. The results indicated a significant increase in lipid droplet size and number after treatment with monoolein formulations compared to untreated controls. Specifically, there was a notable increase in integrated optical density (IOD) per cell, indicating enhanced lipid accumulation .
- The study measured mitochondrial membrane potential (mitoMP) changes, revealing that cubosome-treated cells exhibited a significant hyperpolarization compared to controls. This hyperpolarization was associated with increased ROS generation, suggesting a complex interaction between lipid metabolism and mitochondrial function .
-
Drug Delivery Applications :
- Monoolein has been explored as a carrier for enhancing the solubility and bioavailability of poorly soluble drugs. Research demonstrated that monoolein-loaded cubosomes significantly improved the pharmacokinetic profiles of encapsulated drugs, leading to higher systemic exposure compared to conventional formulations .
Data Table: Summary of Key Research Findings
特性
IUPAC Name |
1,3-dihydroxypropan-2-yl (Z)-octadec-9-enoate | |
---|---|---|
Source | PubChem | |
URL | https://pubchem.ncbi.nlm.nih.gov | |
Description | Data deposited in or computed by PubChem | |
InChI |
InChI=1S/C21H40O4/c1-2-3-4-5-6-7-8-9-10-11-12-13-14-15-16-17-21(24)25-20(18-22)19-23/h9-10,20,22-23H,2-8,11-19H2,1H3/b10-9- | |
Source | PubChem | |
URL | https://pubchem.ncbi.nlm.nih.gov | |
Description | Data deposited in or computed by PubChem | |
InChI Key |
UPWGQKDVAURUGE-KTKRTIGZSA-N | |
Source | PubChem | |
URL | https://pubchem.ncbi.nlm.nih.gov | |
Description | Data deposited in or computed by PubChem | |
Canonical SMILES |
CCCCCCCCC=CCCCCCCCC(=O)OC(CO)CO | |
Source | PubChem | |
URL | https://pubchem.ncbi.nlm.nih.gov | |
Description | Data deposited in or computed by PubChem | |
Isomeric SMILES |
CCCCCCCC/C=C\CCCCCCCC(=O)OC(CO)CO | |
Source | PubChem | |
URL | https://pubchem.ncbi.nlm.nih.gov | |
Description | Data deposited in or computed by PubChem | |
Molecular Formula |
C21H40O4 | |
Source | PubChem | |
URL | https://pubchem.ncbi.nlm.nih.gov | |
Description | Data deposited in or computed by PubChem | |
DSSTOX Substance ID |
DTXSID8058661 | |
Record name | 2-Oleoylglycerol | |
Source | EPA DSSTox | |
URL | https://comptox.epa.gov/dashboard/DTXSID8058661 | |
Description | DSSTox provides a high quality public chemistry resource for supporting improved predictive toxicology. | |
Molecular Weight |
356.5 g/mol | |
Source | PubChem | |
URL | https://pubchem.ncbi.nlm.nih.gov | |
Description | Data deposited in or computed by PubChem | |
Physical Description |
Solid | |
Record name | MG(0:0/18:1(9Z)/0:0) | |
Source | Human Metabolome Database (HMDB) | |
URL | http://www.hmdb.ca/metabolites/HMDB0011537 | |
Description | The Human Metabolome Database (HMDB) is a freely available electronic database containing detailed information about small molecule metabolites found in the human body. | |
Explanation | HMDB is offered to the public as a freely available resource. Use and re-distribution of the data, in whole or in part, for commercial purposes requires explicit permission of the authors and explicit acknowledgment of the source material (HMDB) and the original publication (see the HMDB citing page). We ask that users who download significant portions of the database cite the HMDB paper in any resulting publications. | |
CAS No. |
3443-84-3 | |
Record name | 2-Oleoylglycerol | |
Source | CAS Common Chemistry | |
URL | https://commonchemistry.cas.org/detail?cas_rn=3443-84-3 | |
Description | CAS Common Chemistry is an open community resource for accessing chemical information. Nearly 500,000 chemical substances from CAS REGISTRY cover areas of community interest, including common and frequently regulated chemicals, and those relevant to high school and undergraduate chemistry classes. This chemical information, curated by our expert scientists, is provided in alignment with our mission as a division of the American Chemical Society. | |
Explanation | The data from CAS Common Chemistry is provided under a CC-BY-NC 4.0 license, unless otherwise stated. | |
Record name | 2-Glyceryl monooleate | |
Source | ChemIDplus | |
URL | https://pubchem.ncbi.nlm.nih.gov/substance/?source=chemidplus&sourceid=0003443843 | |
Description | ChemIDplus is a free, web search system that provides access to the structure and nomenclature authority files used for the identification of chemical substances cited in National Library of Medicine (NLM) databases, including the TOXNET system. | |
Record name | 2-Oleoylglycerol | |
Source | EPA DSSTox | |
URL | https://comptox.epa.gov/dashboard/DTXSID8058661 | |
Description | DSSTox provides a high quality public chemistry resource for supporting improved predictive toxicology. | |
Record name | GLYCERYL 2-OLEATE | |
Source | FDA Global Substance Registration System (GSRS) | |
URL | https://gsrs.ncats.nih.gov/ginas/app/beta/substances/9A2389K694 | |
Description | The FDA Global Substance Registration System (GSRS) enables the efficient and accurate exchange of information on what substances are in regulated products. Instead of relying on names, which vary across regulatory domains, countries, and regions, the GSRS knowledge base makes it possible for substances to be defined by standardized, scientific descriptions. | |
Explanation | Unless otherwise noted, the contents of the FDA website (www.fda.gov), both text and graphics, are not copyrighted. They are in the public domain and may be republished, reprinted and otherwise used freely by anyone without the need to obtain permission from FDA. Credit to the U.S. Food and Drug Administration as the source is appreciated but not required. | |
Retrosynthesis Analysis
AI-Powered Synthesis Planning: Our tool employs the Template_relevance Pistachio, Template_relevance Bkms_metabolic, Template_relevance Pistachio_ringbreaker, Template_relevance Reaxys, Template_relevance Reaxys_biocatalysis model, leveraging a vast database of chemical reactions to predict feasible synthetic routes.
One-Step Synthesis Focus: Specifically designed for one-step synthesis, it provides concise and direct routes for your target compounds, streamlining the synthesis process.
Accurate Predictions: Utilizing the extensive PISTACHIO, BKMS_METABOLIC, PISTACHIO_RINGBREAKER, REAXYS, REAXYS_BIOCATALYSIS database, our tool offers high-accuracy predictions, reflecting the latest in chemical research and data.
Strategy Settings
Precursor scoring | Relevance Heuristic |
---|---|
Min. plausibility | 0.01 |
Model | Template_relevance |
Template Set | Pistachio/Bkms_metabolic/Pistachio_ringbreaker/Reaxys/Reaxys_biocatalysis |
Top-N result to add to graph | 6 |
Feasible Synthetic Routes
試験管内研究製品の免責事項と情報
BenchChemで提示されるすべての記事および製品情報は、情報提供を目的としています。BenchChemで購入可能な製品は、生体外研究のために特別に設計されています。生体外研究は、ラテン語の "in glass" に由来し、生物体の外で行われる実験を指します。これらの製品は医薬品または薬として分類されておらず、FDAから任何の医療状態、病気、または疾患の予防、治療、または治癒のために承認されていません。これらの製品を人間または動物に体内に導入する形態は、法律により厳格に禁止されています。これらのガイドラインに従うことは、研究と実験において法的および倫理的な基準の遵守を確実にするために重要です。