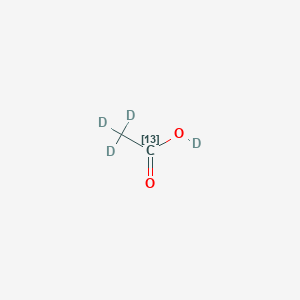
Deuterio 2,2,2-trideuterioacetate
説明
Deuterio 2,2,2-trideuterioacetate, also known as Acetic-d3 acid-d or Acetic Acid-d4, is a chemical reagent used in various applications . It has a molecular formula of C2D4O2 and a molecular weight of 64.077 .
Molecular Structure Analysis
The molecular structure of Deuterio 2,2,2-trideuterioacetate consists of two carbon atoms, four deuterium atoms, and two oxygen atoms . The exact mass is 64.046234 .
Physical And Chemical Properties Analysis
Deuterio 2,2,2-trideuterioacetate has a density of 1.1±0.1 g/cm3, a boiling point of 117.1±3.0 °C at 760 mmHg, and a melting point of 15-16ºC . It has a flash point of 40.0±0.0 °C . The vapour density is 2.07 (vs air), and the vapour pressure is 13.9±0.2 mmHg at 25°C . The index of refraction is 1.376 .
科学的研究の応用
Pharmacokinetics and Metabolic Profiles
Deuterium Substitution Impact : Deuterium substitution in drug molecules can alter their pharmacokinetic and metabolic profiles, potentially offering advantages over nondeuterated forms, including alterations in clearance and redirection of metabolic pathways to reduce toxicities (Russak & Bednarczyk, 2018).
Precision Deuteration : Deuteration in medicinal chemistry can address issues like metabolism-mediated toxicity, drug interactions, and low bioactivation, extending beyond simply improving pharmacokinetic parameters. It can also lower the degree of epimerization and reduce the dosage of coadministered boosters (Pirali, Serafini, Cargnin, & Genazzani, 2019).
Drug Discovery and Development
Deuterated Building Blocks : The preparation of stereoselectively deuterated building blocks for pharmaceutical research can help improve the pharmacological and toxicological properties of drugs. This approach allows for precise control over deuterium incorporation, influencing drug efficacy and safety (Smith et al., 2020).
Deuterated Drug Molecules : Deuterated drugs can retain their biochemical potency and selectivity while showing differentiated pharmacokinetic profiles compared to their hydrogen-only counterparts. This use of deuterium is a powerful tool in medicinal chemistry for improving drug properties (Harbeson & TungRoger, 2011).
Specific Application Examples
Photoredox-catalyzed Deuteration : A photoredox-mediated protocol for deuterium labeling of drug molecules facilitates tracking their biological fate and contributes to drug metabolism studies (Loh et al., 2017).
Raman Spectrum Analysis : The study of the Raman spectrum of Deuterio 2,2,2-trideuterioacetate (CD3CO2D) has provided valuable insights, particularly in the field of chemical analysis and spectroscopy (Angus, Leckie, & Wilson, 1935).
Deuterium in Drug Discovery : Deuteration's utility in mechanistic, spectroscopic, and tracer studies within various subdisciplines of pharmaceutical discovery and development highlights its versatility and potential benefits in altering drug action (Gant, 2014).
Safety And Hazards
Deuterio 2,2,2-trideuterioacetate is classified as a flammable liquid and vapour. It causes severe skin burns and eye damage . Safety measures include keeping away from heat, sparks, open flames, and other ignition sources. In case of contact with eyes, rinse cautiously with water for several minutes and immediately call a poison center or doctor .
特性
IUPAC Name |
deuterio 2,2,2-trideuterioacetate | |
---|---|---|
Source | PubChem | |
URL | https://pubchem.ncbi.nlm.nih.gov | |
Description | Data deposited in or computed by PubChem | |
InChI |
InChI=1S/C2H4O2/c1-2(3)4/h1H3,(H,3,4)/i1D3,2+1/hD | |
Source | PubChem | |
URL | https://pubchem.ncbi.nlm.nih.gov | |
Description | Data deposited in or computed by PubChem | |
InChI Key |
QTBSBXVTEAMEQO-OIFRIMMXSA-N | |
Source | PubChem | |
URL | https://pubchem.ncbi.nlm.nih.gov | |
Description | Data deposited in or computed by PubChem | |
Canonical SMILES |
CC(=O)O | |
Source | PubChem | |
URL | https://pubchem.ncbi.nlm.nih.gov | |
Description | Data deposited in or computed by PubChem | |
Isomeric SMILES |
[2H]C([2H])([2H])[13C](=O)O[2H] | |
Source | PubChem | |
URL | https://pubchem.ncbi.nlm.nih.gov | |
Description | Data deposited in or computed by PubChem | |
Molecular Formula |
C2H4O2 | |
Source | PubChem | |
URL | https://pubchem.ncbi.nlm.nih.gov | |
Description | Data deposited in or computed by PubChem | |
DSSTOX Substance ID |
DTXSID40583509 | |
Record name | (1-~13~C,~2~H_4_)Acetic acid | |
Source | EPA DSSTox | |
URL | https://comptox.epa.gov/dashboard/DTXSID40583509 | |
Description | DSSTox provides a high quality public chemistry resource for supporting improved predictive toxicology. | |
Molecular Weight |
65.069 g/mol | |
Source | PubChem | |
URL | https://pubchem.ncbi.nlm.nih.gov | |
Description | Data deposited in or computed by PubChem | |
Product Name |
Deuterio 2,2,2-trideuterioacetate | |
CAS RN |
63459-47-2 | |
Record name | (1-~13~C,~2~H_4_)Acetic acid | |
Source | EPA DSSTox | |
URL | https://comptox.epa.gov/dashboard/DTXSID40583509 | |
Description | DSSTox provides a high quality public chemistry resource for supporting improved predictive toxicology. | |
Record name | Acetic acid-1-13C,d4 | |
Source | European Chemicals Agency (ECHA) | |
URL | https://echa.europa.eu/information-on-chemicals | |
Description | The European Chemicals Agency (ECHA) is an agency of the European Union which is the driving force among regulatory authorities in implementing the EU's groundbreaking chemicals legislation for the benefit of human health and the environment as well as for innovation and competitiveness. | |
Explanation | Use of the information, documents and data from the ECHA website is subject to the terms and conditions of this Legal Notice, and subject to other binding limitations provided for under applicable law, the information, documents and data made available on the ECHA website may be reproduced, distributed and/or used, totally or in part, for non-commercial purposes provided that ECHA is acknowledged as the source: "Source: European Chemicals Agency, http://echa.europa.eu/". Such acknowledgement must be included in each copy of the material. ECHA permits and encourages organisations and individuals to create links to the ECHA website under the following cumulative conditions: Links can only be made to webpages that provide a link to the Legal Notice page. | |
試験管内研究製品の免責事項と情報
BenchChemで提示されるすべての記事および製品情報は、情報提供を目的としています。BenchChemで購入可能な製品は、生体外研究のために特別に設計されています。生体外研究は、ラテン語の "in glass" に由来し、生物体の外で行われる実験を指します。これらの製品は医薬品または薬として分類されておらず、FDAから任何の医療状態、病気、または疾患の予防、治療、または治癒のために承認されていません。これらの製品を人間または動物に体内に導入する形態は、法律により厳格に禁止されています。これらのガイドラインに従うことは、研究と実験において法的および倫理的な基準の遵守を確実にするために重要です。