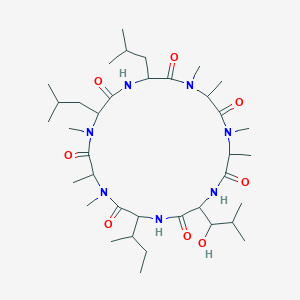
テルナチンヘプタペプチド
概要
説明
テルナチンは、いくつかの菌種によって産生される天然の環状ペプチドですテルナチンは、伸長因子-1A三元複合体を標的としてタンパク質合成を阻害する能力で知られており、治療開発のための有望な候補です .
2. 製法
合成経路と反応条件: テルナチンとその合成変異体は、固相ペプチド合成(SPPS)によって調製できます。この方法は、固体樹脂に固定された成長するペプチド鎖にアミノ酸を逐次的に付加することを含みます。このプロセスには、脱保護とカップリングのステップが含まれ、目的のペプチド配列が得られるまで繰り返されます。 最終生成物は、その後樹脂から切断され、精製されます .
工業生産方法: テルナチンの工業生産には、この化合物を自然に生成する菌類を用いた発酵プロセスが含まれます。菌類は、テルナチンの収量を最適化するために、制御された条件下で大型バイオリアクターで培養されます。 その後、この化合物は、クロマトグラフィー技術を使用して抽出および精製されます .
科学的研究の応用
Ternatin has a wide range of scientific research applications, including:
Chemistry: Ternatin serves as a valuable chemical probe for studying protein synthesis and translation elongation.
Biology: It is used to investigate the mechanisms of protein translation and the role of elongation factor-1A in cellular processes.
Medicine: Ternatin and its derivatives are being explored as potential anticancer agents due to their ability to inhibit protein synthesis in cancer cells.
Industry: Ternatin’s unique properties make it a candidate for developing new therapeutic drugs and chemical probes
作用機序
テルナチンは、伸長因子-1A三元複合体(eEF1A・GTP・アミノアシルtRNA)に結合することでその効果を発揮します。この結合は、eEF1Aの正常な機能を阻害し、タンパク質合成中の新生ポリペプチド鎖の適切な伸長を妨げます。 その結果、タンパク質翻訳が阻害され、細胞に細胞毒性効果をもたらします .
類似化合物:
ディデムニン: 伸長因子-1A三元複合体を標的とする別の環状ペプチドですが、異なる動態特性を示します。
シトトリエニン: eEF1Aに結合し、タンパク質合成を阻害する天然物。
テルナチンの独自性: テルナチンは、eEF1A三元複合体に対する特異的な結合親和性、およびeEF1Aの分解を誘導する能力のためにユニークです。 この分解は、ディデムニンなどの他の類似化合物では観察されず、テルナチンは治療開発のための独特で貴重な化合物となっています .
将来の方向性
生化学分析
Biochemical Properties
Ternatin heptapeptide disrupts a central biological process, protein translation, by binding to the translation elongation factor 1A . This protein participates in a carefully orchestrated series of reactions with guanine nucleotides, transfer RNAs (tRNAs), a guanine nucleotide exchange factor, and the elongating ribosome . The nature of these interactions is complex and involves a multitude of biomolecules.
Cellular Effects
Ternatin heptapeptide has been found to have significant effects on various types of cells. It is cytotoxic toward cancer cells, with synthetic variants showing up to 500-fold greater potency than Ternatin itself . It inhibits protein synthesis in cells, which correlates with its ability to block cell proliferation .
Molecular Mechanism
The molecular mechanism of action of Ternatin heptapeptide involves its binding to the translation elongation factor-1A ternary complex (eEF1A·GTP·aminoacyl-tRNA) as a specific target . Mutations in domain III of eEF1A prevent Ternatin binding and confer resistance to its cytotoxic effects, implicating the adjacent hydrophobic surface as a functional hot spot for eEF1A modulation .
Temporal Effects in Laboratory Settings
It has been observed that Ternatin can kill mammalian cells in high doses
Dosage Effects in Animal Models
The effects of Ternatin heptapeptide at different dosages in animal models have not been extensively studied. It is known that Ternatin can kill mammalian cells in high doses
Metabolic Pathways
Ternatin heptapeptide is involved in the protein translation pathway, specifically by binding to the translation elongation factor 1A This interaction disrupts the normal function of this pathway
Transport and Distribution
Given its role in protein translation, it is likely that it interacts with various transporters and binding proteins involved in this process .
Subcellular Localization
Given its role in protein translation, it is likely that it is localized to the ribosomes where protein synthesis occurs
準備方法
Synthetic Routes and Reaction Conditions: Ternatin and its synthetic variants can be prepared through solid-phase peptide synthesis (SPPS). This method involves the sequential addition of amino acids to a growing peptide chain anchored to a solid resin. The process includes deprotection and coupling steps, which are repeated until the desired peptide sequence is obtained. The final product is then cleaved from the resin and purified .
Industrial Production Methods: Industrial production of ternatin involves fermentation processes using fungi that naturally produce the compound. The fungi are cultured in large bioreactors under controlled conditions to optimize the yield of ternatin. The compound is then extracted and purified using chromatographic techniques .
化学反応の分析
反応の種類: テルナチンは、以下のものを含むさまざまな化学反応を受けます。
酸化: テルナチンは、酸化されて、生物学的活性が変化したさまざまな誘導体を形成することができます。
還元: 還元反応は、テルナチン内のペプチド結合を変性させることができ、その安定性と活性に影響を与える可能性があります。
一般的な試薬と条件:
酸化: 一般的な酸化剤には、過酸化水素と過マンガン酸カリウムが含まれます。
還元: 水素化ホウ素ナトリウムや水素化アルミニウムリチウムなどの還元剤が使用されます。
生成される主な生成物: これらの反応から生成される主な生成物には、細胞毒性が強化され、薬物動態特性が改善された、さまざまなテルナチン誘導体が含まれます .
4. 科学研究における用途
テルナチンは、以下を含む幅広い科学研究用途を持っています。
化学: テルナチンは、タンパク質合成と翻訳伸長を研究するための貴重な化学プローブとして役立ちます。
生物学: タンパク質翻訳のメカニズム、および細胞プロセスにおける伸長因子-1Aの役割を調査するために使用されます。
医学: テルナチンとその誘導体は、癌細胞でのタンパク質合成を阻害する能力のために、潜在的な抗癌剤として研究されています。
類似化合物との比較
Didemnin: Another cyclic peptide that targets the elongation factor-1A ternary complex but exhibits different kinetic properties.
Cytotrienin: A natural product that also binds to eEF1A and inhibits protein synthesis.
Uniqueness of Ternatin: Ternatin is unique due to its specific binding affinity for the eEF1A ternary complex and its ability to induce degradation of eEF1A. This degradation is not observed with other similar compounds like didemnin, making ternatin a distinct and valuable compound for therapeutic development .
特性
IUPAC Name |
(3S,6S,9S,12S,15R,18R,21R)-15-[(2S)-butan-2-yl]-18-[(1R)-1-hydroxy-2-methylpropyl]-1,3,4,10,12,13,21-heptamethyl-6,9-bis(2-methylpropyl)-1,4,7,10,13,16,19-heptazacyclohenicosane-2,5,8,11,14,17,20-heptone | |
---|---|---|
Details | Computed by LexiChem 2.6.6 (PubChem release 2019.06.18) | |
Source | PubChem | |
URL | https://pubchem.ncbi.nlm.nih.gov | |
Description | Data deposited in or computed by PubChem | |
InChI |
InChI=1S/C37H67N7O8/c1-16-22(8)28-37(52)43(14)25(11)35(50)44(15)27(18-20(4)5)32(47)38-26(17-19(2)3)36(51)42(13)24(10)34(49)41(12)23(9)31(46)40-29(33(48)39-28)30(45)21(6)7/h19-30,45H,16-18H2,1-15H3,(H,38,47)(H,39,48)(H,40,46)/t22-,23+,24-,25-,26-,27-,28+,29+,30+/m0/s1 | |
Details | Computed by InChI 1.0.5 (PubChem release 2019.06.18) | |
Source | PubChem | |
URL | https://pubchem.ncbi.nlm.nih.gov | |
Description | Data deposited in or computed by PubChem | |
InChI Key |
ZMFVAIFXJWEOMH-PTPSPKLBSA-N | |
Details | Computed by InChI 1.0.5 (PubChem release 2019.06.18) | |
Source | PubChem | |
URL | https://pubchem.ncbi.nlm.nih.gov | |
Description | Data deposited in or computed by PubChem | |
Canonical SMILES |
CCC(C)C1C(=O)N(C(C(=O)N(C(C(=O)NC(C(=O)N(C(C(=O)N(C(C(=O)NC(C(=O)N1)C(C(C)C)O)C)C)C)C)CC(C)C)CC(C)C)C)C)C | |
Details | Computed by OEChem 2.1.5 (PubChem release 2019.06.18) | |
Source | PubChem | |
URL | https://pubchem.ncbi.nlm.nih.gov | |
Description | Data deposited in or computed by PubChem | |
Isomeric SMILES |
CC[C@H](C)[C@@H]1C(=O)N([C@H](C(=O)N([C@H](C(=O)N[C@H](C(=O)N([C@H](C(=O)N([C@@H](C(=O)N[C@@H](C(=O)N1)[C@@H](C(C)C)O)C)C)C)C)CC(C)C)CC(C)C)C)C)C | |
Details | Computed by OEChem 2.1.5 (PubChem release 2019.06.18) | |
Source | PubChem | |
URL | https://pubchem.ncbi.nlm.nih.gov | |
Description | Data deposited in or computed by PubChem | |
Molecular Formula |
C37H67N7O8 | |
Details | Computed by PubChem 2.1 (PubChem release 2019.06.18) | |
Source | PubChem | |
URL | https://pubchem.ncbi.nlm.nih.gov | |
Description | Data deposited in or computed by PubChem | |
DSSTOX Substance ID |
DTXSID20933442 | |
Record name | Ternatin | |
Source | EPA DSSTox | |
URL | https://comptox.epa.gov/dashboard/DTXSID20933442 | |
Description | DSSTox provides a high quality public chemistry resource for supporting improved predictive toxicology. | |
Molecular Weight |
738.0 g/mol | |
Details | Computed by PubChem 2.1 (PubChem release 2021.05.07) | |
Source | PubChem | |
URL | https://pubchem.ncbi.nlm.nih.gov | |
Description | Data deposited in or computed by PubChem | |
CAS No. |
148619-41-4 | |
Record name | Ternatin | |
Source | EPA DSSTox | |
URL | https://comptox.epa.gov/dashboard/DTXSID20933442 | |
Description | DSSTox provides a high quality public chemistry resource for supporting improved predictive toxicology. | |
Retrosynthesis Analysis
AI-Powered Synthesis Planning: Our tool employs the Template_relevance Pistachio, Template_relevance Bkms_metabolic, Template_relevance Pistachio_ringbreaker, Template_relevance Reaxys, Template_relevance Reaxys_biocatalysis model, leveraging a vast database of chemical reactions to predict feasible synthetic routes.
One-Step Synthesis Focus: Specifically designed for one-step synthesis, it provides concise and direct routes for your target compounds, streamlining the synthesis process.
Accurate Predictions: Utilizing the extensive PISTACHIO, BKMS_METABOLIC, PISTACHIO_RINGBREAKER, REAXYS, REAXYS_BIOCATALYSIS database, our tool offers high-accuracy predictions, reflecting the latest in chemical research and data.
Strategy Settings
Precursor scoring | Relevance Heuristic |
---|---|
Min. plausibility | 0.01 |
Model | Template_relevance |
Template Set | Pistachio/Bkms_metabolic/Pistachio_ringbreaker/Reaxys/Reaxys_biocatalysis |
Top-N result to add to graph | 6 |
Feasible Synthetic Routes
A: Ternatin targets the eukaryotic elongation factor-1A (eEF1A) ternary complex, specifically the eEF1A·GTP·aminoacyl-tRNA complex. [, , ] This complex plays a crucial role in protein synthesis by delivering aminoacyl-tRNAs to the ribosome.
A: Ternatin binding traps eEF1A in an intermediate state of aminoacyl-tRNA selection, preventing its release and subsequent accommodation of the aminoacyl-tRNA into the ribosome. [, ] This effectively halts protein translation.
A: Yes, studies have shown that ternatin induces the degradation of eEF1A, a phenomenon not observed with other eEF1A inhibitors like didemnin B. [, ] This degradation appears to involve a ubiquitin-dependent pathway and may be linked to ribosome-associated quality control mechanisms.
A: By inhibiting eEF1A and disrupting protein synthesis, ternatin exhibits cytotoxic effects, particularly against cancer cells. [, ] This has sparked interest in its potential as an anticancer agent.
A: Ternatin is a cyclic heptapeptide. Its molecular formula is C38H65N7O9 and its molecular weight is 763.96 g/mol. []
A: The presence of four N-methylated amino acid residues in ternatin's structure appears to restrict the number of low-energy conformations the molecule can adopt. [] This conformational rigidity may contribute to its specific biological activity.
A: Ternatin is characterized by a beta-turn structure stabilized by intramolecular hydrogen bonds. [] This structural feature has been shown to be essential for its fat-accumulation inhibitory activity.
ANone: The provided research focuses on ternatin's biological activity and does not offer insights into material compatibility or stability relevant for material science applications.
ANone: Ternatin is not reported to have catalytic properties. Its mechanism of action primarily involves binding and inhibiting a biological target, eEF1A, rather than catalyzing a chemical reaction.
A: Yes, molecular docking simulations have been employed to explore ternatin's interactions with potential targets, including cholera toxin and SARS-CoV-2 nucleocapsid protein. [, ] These studies provide insights into ternatin's binding affinities and potential therapeutic applications.
A: While the provided research does not mention specific QSAR studies on ternatin, one study utilized molecular modeling to analyze ternatin's electronic and structural characteristics, laying the groundwork for future drug design and QSAR investigations. []
A: Studies focusing on the beta-OH-D-Leu moiety of ternatin have demonstrated the importance of its backbone conformation for fat-accumulation inhibitory activity. [, ] Modifications that disrupt this conformation could potentially diminish its efficacy.
A: Systematic replacement of each amino acid in ternatin with alanine revealed that the D-Ile residue plays a crucial role in its biological activity. [] This finding highlights the importance of specific amino acid residues in maintaining ternatin's potency.
試験管内研究製品の免責事項と情報
BenchChemで提示されるすべての記事および製品情報は、情報提供を目的としています。BenchChemで購入可能な製品は、生体外研究のために特別に設計されています。生体外研究は、ラテン語の "in glass" に由来し、生物体の外で行われる実験を指します。これらの製品は医薬品または薬として分類されておらず、FDAから任何の医療状態、病気、または疾患の予防、治療、または治癒のために承認されていません。これらの製品を人間または動物に体内に導入する形態は、法律により厳格に禁止されています。これらのガイドラインに従うことは、研究と実験において法的および倫理的な基準の遵守を確実にするために重要です。