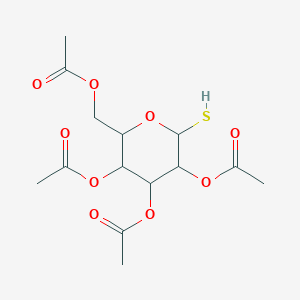
1-Thio-beta-D-glucose tetraacetate
概要
説明
1-Thio-beta-D-glucose tetraacetate, also known as 2,3,4,6-Tetra-O-acetyl-1-thio-beta-D-glucopyranose, is a chemical compound with the molecular formula C14H20O9S. It is a derivative of glucose where the hydroxyl group at the anomeric carbon is replaced by a thiol group, and the remaining hydroxyl groups are acetylated. This compound is used in various chemical and biological applications due to its unique properties .
準備方法
1-Thio-beta-D-glucose tetraacetate can be synthesized through several methods. One common synthetic route involves the acetylation of 1-thio-beta-D-glucose. The reaction typically uses acetic anhydride in the presence of a catalyst such as pyridine. The reaction conditions include maintaining the temperature at around 0-5°C to control the reaction rate and prevent side reactions .
Industrial production methods may involve similar acetylation processes but on a larger scale, with optimized reaction conditions to ensure high yield and purity. The use of continuous flow reactors and advanced purification techniques such as chromatography can enhance the efficiency of industrial production .
化学反応の分析
1-Thio-beta-D-glucose tetraacetate undergoes various chemical reactions, including:
Oxidation: The thiol group can be oxidized to form disulfides or sulfonic acids. Common oxidizing agents include hydrogen peroxide and sodium periodate.
Reduction: The compound can be reduced to form the corresponding alcohol. Reducing agents such as lithium aluminum hydride (LiAlH4) are commonly used.
Substitution: The acetyl groups can be substituted with other functional groups through nucleophilic substitution reactions.
The major products formed from these reactions depend on the specific reagents and conditions used. For instance, oxidation with hydrogen peroxide can yield sulfonic acids, while reduction with LiAlH4 can produce the corresponding alcohol .
科学的研究の応用
作用機序
The mechanism of action of 1-Thio-beta-D-glucose tetraacetate involves its interaction with various molecular targets. The thiol group can form covalent bonds with proteins and other biomolecules, affecting their function. This property is exploited in its use as an inhibitor of the Maillard reaction, where it prevents the formation of advanced glycation end-products (AGEs) by reacting with reactive carbonyl groups .
類似化合物との比較
1-Thio-beta-D-glucose tetraacetate can be compared with other similar compounds such as:
1-Thio-beta-D-glucose sodium salt: This compound has a similar structure but lacks the acetyl groups, making it more soluble in water and suitable for different applications.
5-Thio-D-glucose: Another thiol derivative of glucose, but with the thiol group at a different position, leading to different reactivity and applications.
Acetobromo-alpha-D-glucose: This compound has a bromine atom instead of a thiol group, making it useful in different types of substitution reactions.
The uniqueness of this compound lies in its combination of a thiol group and acetylated hydroxyl groups, providing a balance of reactivity and stability that is valuable in various chemical and biological contexts .
特性
IUPAC Name |
(3,4,5-triacetyloxy-6-sulfanyloxan-2-yl)methyl acetate | |
---|---|---|
Details | Computed by Lexichem TK 2.7.0 (PubChem release 2021.05.07) | |
Source | PubChem | |
URL | https://pubchem.ncbi.nlm.nih.gov | |
Description | Data deposited in or computed by PubChem | |
InChI |
InChI=1S/C14H20O9S/c1-6(15)19-5-10-11(20-7(2)16)12(21-8(3)17)13(14(24)23-10)22-9(4)18/h10-14,24H,5H2,1-4H3 | |
Details | Computed by InChI 1.0.6 (PubChem release 2021.05.07) | |
Source | PubChem | |
URL | https://pubchem.ncbi.nlm.nih.gov | |
Description | Data deposited in or computed by PubChem | |
InChI Key |
SFOZKJGZNOBSHF-UHFFFAOYSA-N | |
Details | Computed by InChI 1.0.6 (PubChem release 2021.05.07) | |
Source | PubChem | |
URL | https://pubchem.ncbi.nlm.nih.gov | |
Description | Data deposited in or computed by PubChem | |
Canonical SMILES |
CC(=O)OCC1C(C(C(C(O1)S)OC(=O)C)OC(=O)C)OC(=O)C | |
Details | Computed by OEChem 2.3.0 (PubChem release 2021.05.07) | |
Source | PubChem | |
URL | https://pubchem.ncbi.nlm.nih.gov | |
Description | Data deposited in or computed by PubChem | |
Molecular Formula |
C14H20O9S | |
Details | Computed by PubChem 2.1 (PubChem release 2021.05.07) | |
Source | PubChem | |
URL | https://pubchem.ncbi.nlm.nih.gov | |
Description | Data deposited in or computed by PubChem | |
Molecular Weight |
364.37 g/mol | |
Details | Computed by PubChem 2.1 (PubChem release 2021.05.07) | |
Source | PubChem | |
URL | https://pubchem.ncbi.nlm.nih.gov | |
Description | Data deposited in or computed by PubChem | |
CAS No. |
19879-84-6 | |
Record name | NSC97032 | |
Source | DTP/NCI | |
URL | https://dtp.cancer.gov/dtpstandard/servlet/dwindex?searchtype=NSC&outputformat=html&searchlist=97032 | |
Description | The NCI Development Therapeutics Program (DTP) provides services and resources to the academic and private-sector research communities worldwide to facilitate the discovery and development of new cancer therapeutic agents. | |
Explanation | Unless otherwise indicated, all text within NCI products is free of copyright and may be reused without our permission. Credit the National Cancer Institute as the source. | |
Retrosynthesis Analysis
AI-Powered Synthesis Planning: Our tool employs the Template_relevance Pistachio, Template_relevance Bkms_metabolic, Template_relevance Pistachio_ringbreaker, Template_relevance Reaxys, Template_relevance Reaxys_biocatalysis model, leveraging a vast database of chemical reactions to predict feasible synthetic routes.
One-Step Synthesis Focus: Specifically designed for one-step synthesis, it provides concise and direct routes for your target compounds, streamlining the synthesis process.
Accurate Predictions: Utilizing the extensive PISTACHIO, BKMS_METABOLIC, PISTACHIO_RINGBREAKER, REAXYS, REAXYS_BIOCATALYSIS database, our tool offers high-accuracy predictions, reflecting the latest in chemical research and data.
Strategy Settings
Precursor scoring | Relevance Heuristic |
---|---|
Min. plausibility | 0.01 |
Model | Template_relevance |
Template Set | Pistachio/Bkms_metabolic/Pistachio_ringbreaker/Reaxys/Reaxys_biocatalysis |
Top-N result to add to graph | 6 |
Feasible Synthetic Routes
Q1: What is the role of 1-thio-beta-D-glucose 2,3,4,6-tetraacetate in the synthesis of the gold complexes described in the paper?
A1: 1-thio-beta-D-glucose 2,3,4,6-tetraacetate (SRH) is used as a reagent to introduce a thiolate ligand (SR) to a gold(III) complex. Specifically, it reacts with the complex [(CQ)Au(Cl)(2)]Cl (where CQ is chloroquine) to form [(CQ)Au(Cl)(SR)(Et(2)O)]Cl []. This suggests that the researchers were interested in investigating the impact of modifying the gold(III) complex with a thiolate ligand on its antimalarial activity.
試験管内研究製品の免責事項と情報
BenchChemで提示されるすべての記事および製品情報は、情報提供を目的としています。BenchChemで購入可能な製品は、生体外研究のために特別に設計されています。生体外研究は、ラテン語の "in glass" に由来し、生物体の外で行われる実験を指します。これらの製品は医薬品または薬として分類されておらず、FDAから任何の医療状態、病気、または疾患の予防、治療、または治癒のために承認されていません。これらの製品を人間または動物に体内に導入する形態は、法律により厳格に禁止されています。これらのガイドラインに従うことは、研究と実験において法的および倫理的な基準の遵守を確実にするために重要です。