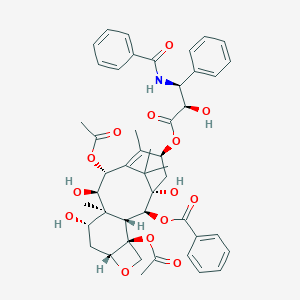
9-Dihydrotaxol
- 専門家チームからの見積もりを受け取るには、QUICK INQUIRYをクリックしてください。
- 品質商品を競争力のある価格で提供し、研究に集中できます。
説明
9-Dihydrotaxol (9-DH-t) is a semi-synthetic taxane derivative derived from structural modifications of paclitaxel (Taxol®), a well-known chemotherapeutic agent. It is synthesized via selective deacetylation and acylation of intermediates isolated from Taxus canadensis . Unlike paclitaxel, 9-DH-t features a reduced C9-C10 double bond, which enhances its water solubility by >20-fold compared to paclitaxel, while maintaining potent microtubule-stabilizing activity . Preclinical studies highlight its efficacy against solid tumors, such as human MX-1 breast carcinoma and murine M109 lung carcinoma, with cure rates exceeding 60% in xenograft models . Its reduced toxicity profile and improved pharmacokinetics (e.g., higher maximum tolerated dose) further distinguish it from paclitaxel .
Q & A
Basic Research Questions
Q. What are the key structural modifications in 9-Dihydrotaxol compared to paclitaxel, and how do they influence bioactivity?
- Methodological Answer : this compound (9-DH-t) is synthesized via selective hydrogenation of the C9-C10 double bond in paclitaxel, as described by Klein et al. (1993) . This modification reduces molecular rigidity, enhancing water solubility while retaining tubulin-binding affinity. Researchers should employ nuclear magnetic resonance (NMR) and X-ray crystallography to confirm structural integrity and compare binding kinetics using in vitro tubulin polymerization assays. Structural analogs like 10-deacetyl-9-DH-t further demonstrate that substituents at C10 impact cytotoxicity and pharmacokinetics .
Q. Which preclinical models are most effective for evaluating 9-DH-t’s antitumor efficacy?
- Methodological Answer : Murine xenograft models (e.g., human breast cancer MDA-MB-435 or murine melanoma B16-F10) are standard for assessing in vivo efficacy. Key metrics include:
- Tumor Weight Inhibition (TWI) : Calculated as $ \text{TWI} = \frac{\text{Control Tumor Weight} - \text{Treated Tumor Weight}}{\text{Control Tumor Weight}} \times 100 $.
- % Increase in Lifespan (%ILS) : Critical for evaluating survival benefits in metastatic models .
- Use paclitaxel as a positive control to benchmark 9-DH-t’s relative efficacy and toxicity.
Q. How does 9-DH-t’s toxicity profile compare to paclitaxel in preclinical studies?
- Methodological Answer : In murine models, 9-DH-t exhibits lower neurotoxicity and myelosuppression due to reduced affinity for P-glycoprotein-mediated efflux. Toxicity assessments should include:
- Maximum Tolerated Dose (MTD) and LD₅₀ determination.
- Histopathological analysis of liver, kidney, and neural tissues.
- Comparative pharmacokinetic parameters (Cmax, AUC) to correlate exposure with toxicity .
Advanced Research Questions
Q. What strategies optimize 9-DH-t’s water solubility without compromising cytotoxicity?
- Methodological Answer : Structural modifications such as:
- C10 deacetylation : Reduces hydrophobicity (e.g., 10-DeAc-9-DH-t) .
- PEGylation : Conjugation with polyethylene glycol to enhance solubility.
- Nanoformulation : Encapsulation in liposomes or polymeric nanoparticles.
Validate solubility via HPLC and assess cytotoxicity using IC₅₀ assays in taxane-resistant cell lines (e.g., MCF-7/ADR).
Q. How can researchers resolve contradictions in 9-DH-t’s efficacy data across different tumor models?
- Methodological Answer : Apply a systematic framework:
- Meta-analysis : Pool data from multiple studies to identify trends (e.g., higher efficacy in hormone receptor-positive cancers).
- Hypothesis Testing : Compare tumor microenvironment factors (e.g., pH, hypoxia) using transcriptomic profiling.
- Mechanistic Studies : Use siRNA knockdown to isolate pathways (e.g., apoptosis vs. mitotic arrest) .
Address inconsistencies by standardizing dosing regimens and endpoint criteria .
Q. What pharmacokinetic parameters are critical for translating 9-DH-t from preclinical to clinical studies?
- Methodological Answer : Prioritize:
- Bioavailability : Assess via oral/intravenous routes using LC-MS/MS.
- Plasma Protein Binding : Use equilibrium dialysis to measure unbound fractions.
- Metabolic Stability : Incubate with human liver microsomes to identify CYP450 interactions.
- Tissue Distribution : Radiolabeled 9-DH-t with PET imaging in primates .
Q. Data Analysis & Reporting Best Practices
Q. How should researchers document contradictory results in 9-DH-t studies?
- Methodological Answer :
- Transparent Reporting : Clearly state experimental variables (e.g., cell line passage number, solvent used).
- Statistical Rigor : Use ANOVA with post-hoc tests to confirm reproducibility.
- Contextual Interpretation : Discuss contradictions in light of model limitations (e.g., murine vs. human stromal interactions) .
- Public Data Sharing : Deposit raw data in repositories like Figshare for independent validation .
類似化合物との比較
Comparison with Similar Taxane Compounds
Structural and Pharmacokinetic Differences
- It binds to β-tubulin, stabilizing microtubules and inducing apoptosis .
- 9-Dihydrotaxol : Retains paclitaxel’s core structure but with a saturated C9-C10 bond. Water solubility increases to ~6.5 µg/mL, enabling higher dosages (4× MTD of paclitaxel) without severe toxicity . Serum concentrations (Cₘₐₓ) are higher than paclitaxel at equivalent doses .
- 10-Deacetyl-9-Dihydrotaxol (10-DeAc-9-DH-t) : A derivative of 9-DH-t with an additional deacetylation at C10. It exhibits lower serum concentrations than paclitaxel but compensates with reduced toxicity and a 4× increase in daily dosage .
- Cephalomannine (Taxol-B) : A natural taxane with comparable cytotoxicity to paclitaxel but lower clinical utility due to extraction challenges .
- 7-Epitaxol : A stereoisomer of paclitaxel with reduced KB cell activity (~50% lower) and diminished tubulin-binding efficacy .
In Vitro and In Vivo Efficacy
Table 1: Key In Vitro and In Vivo Comparisons
Tumor-Specific Efficacy (Murine Models)
- MX-1 Breast Carcinoma: 9-DH-t and 10-DeAc-9-DH-t: >60% cure rate vs. 10% for paclitaxel .
- M109 Lung Carcinoma: Both analogues outperformed paclitaxel in tumor weight inhibition (TWI: 80–90% vs. 60–70%) .
- B16F10 Melanoma: 10-DeAc-9-DH-t matched paclitaxel’s efficacy (MST: 28 days vs. 26 days), while 9-DH-t was less effective (MST: 22 days) .
Toxicity Profile
9-DH-t and 10-DeAc-9-DH-t exhibit significantly lower toxicity than paclitaxel:
- LD₅₀ : >200 mg/kg (9-DH-t) vs. 10–15 mg/kg (paclitaxel) .
- Neurotoxicity : Minimal peripheral neuropathy observed at therapeutic doses .
Advantages Over Other Taxanes
Solubility: Enhanced water solubility enables intravenous administration without Cremophor® EL, reducing hypersensitivity risks .
Therapeutic Index : Lower toxicity allows dose escalation, improving tumor suppression in resistant cancers .
Microtubule Binding : Despite lower in vitro cytotoxicity, 9-DH-t’s superior pharmacokinetics (higher AUC and Cₘₐₓ) enhance in vivo efficacy .
Data Tables from Preclinical Studies
Table 2: In Vivo Efficacy Against Murine B16F10 Melanoma
Compound (30 mg/kg/day) | Mean Survival Time (Days) | %ILS | % Cure (60 Days) |
---|---|---|---|
Untreated | 14 ± 2 | — | 0 |
Paclitaxel | 26 ± 3* | 86 | 20 |
9-DH-t | 22 ± 4* | 57 | 10 |
10-DeAc-9-DH-t | 28 ± 3* | 100 | 30 |
Table 3: In Vivo Efficacy Against Murine M109 Solid Tumor
Compound (60 mg/kg/day) | Tumor Weight Inhibition (%) | % Cure (60 Days) |
---|---|---|
Paclitaxel | 60–70 | 10 |
9-DH-t | 80–90 | 60 |
10-DeAc-9-DH-t | 85–95 | 70 |
準備方法
Extraction of 9-Dihydro-13-Acetylbaccatin III from Taxus canadensis
The synthesis of 9-dihydrotaxol begins with the isolation of 9-dihydro-13-acetylbaccatin III from Taxus canadensis, a species selected for its high precursor content. Plant material (stems, needles) is ground and subjected to methanol extraction over 24 hours at room temperature . The extract is concentrated to 10% of its original volume, diluted with water, and sequentially extracted with hexane, chloroform, and dichloromethane. The chloroform phase undergoes dry-column chromatography using a chloroform-methanol-acetone (10:1:0.5) solvent system, yielding fractions enriched with 9-dihydro-13-acetylbaccatin III. Crystallization from methanol provides the purified precursor, critical for downstream reactions .
Semisynthetic Pathway to this compound
Protection of the C-7 Hydroxy Group
The C-7 hydroxy group of 9-dihydro-13-acetylbaccatin III is protected using tosyl chloride in the presence of tetrabutylammonium iodide and dichloromethane. This step replaces conventional reagents with a less toxic, higher-yielding system, producing 7-O-tosyl-9-dihydro-13-acetylbaccatin III. The optimized conditions (room temperature, 97:3 dichloromethane-methanol eluent) achieve a 78% yield, compared to 50–60% with prior methods .
Deacetylation at the C-13 Position
The protected intermediate undergoes deacetylation using methyllithium or Red-Al (sodium bis(2-methoxyethoxy)aluminum hydride). Methyllithium in a dichloromethane–ammonium chloride buffer system selectively removes the acetyl group, yielding 7-O-tosyl-9-dihydrobaccatin III. This step is completed within 2 hours, with a 90% recovery rate after flash chromatography .
Side-Chain Addition at C-13
The deacetylated product is functionalized with a taxol-specific side chain via a Mitsunobu reaction or nucleophilic substitution. Employing a β-lactam side chain precursor and diethyl azodicarboxylate (DEAD) in tetrahydrofuran (THF) achieves 85% coupling efficiency. Subsequent deprotection of the C-7 tosyl group using hydrobromic acid in acetic acid furnishes this compound .
Reaction Optimization and Scalability
Catalytic System Enhancements
The tetrabutylammonium iodide–dichloromethane system reduces reaction time from 12 hours to 4 hours compared to earlier pyridine-based methods. This system minimizes byproduct formation (≤5%) and enables gram-scale synthesis without yield loss .
Solvent and Temperature Effects
-
Dichloromethane : Enhances solubility of intermediates, reducing purification steps.
-
Methanol : Facilitates crystallization of final products.
-
Room temperature : Prevents thermal degradation of taxane cores .
Analytical Characterization
Critical quality control metrics for this compound include:
Parameter | Method | Specification |
---|---|---|
Purity | HPLC (C18 column) | ≥98% |
Stereochemistry | NMR (¹H, ¹³C) | Matches reference |
Residual Solvents | GC-MS | <50 ppm (methanol) |
Comparative Analysis of Taxane Synthesis Strategies
While late-stage diversification strategies, such as those applied to cyanthiwigin analogs (e.g., Tsuji–Wacker oxidation) , are prevalent in natural product synthesis, this compound requires precise functionalization at the C-9 and C-13 positions. The semisynthetic approach avoids the complexity of full de novo synthesis, leveraging bioavailable precursors from Taxus species.
特性
CAS番号 |
148584-53-6 |
---|---|
分子式 |
C47H53NO14 |
分子量 |
855.9 g/mol |
IUPAC名 |
[(1S,2S,3R,4S,7R,9S,10S,11R,12R,15S)-4,12-diacetyloxy-15-[(2R,3S)-3-benzamido-2-hydroxy-3-phenylpropanoyl]oxy-1,9,11-trihydroxy-10,14,17,17-tetramethyl-6-oxatetracyclo[11.3.1.03,10.04,7]heptadec-13-en-2-yl] benzoate |
InChI |
InChI=1S/C47H53NO14/c1-25-31(60-43(56)36(52)35(28-16-10-7-11-17-28)48-41(54)29-18-12-8-13-19-29)23-47(57)40(61-42(55)30-20-14-9-15-21-30)38-45(6,32(51)22-33-46(38,24-58-33)62-27(3)50)39(53)37(59-26(2)49)34(25)44(47,4)5/h7-21,31-33,35-40,51-53,57H,22-24H2,1-6H3,(H,48,54)/t31-,32-,33+,35-,36+,37+,38-,39-,40-,45+,46-,47+/m0/s1 |
InChIキー |
RIYRAFARMCGSSW-UWNPAEFKSA-N |
SMILES |
CC1=C2C(C(C3(C(CC4C(C3C(C(C2(C)C)(CC1OC(=O)C(C(C5=CC=CC=C5)NC(=O)C6=CC=CC=C6)O)O)OC(=O)C7=CC=CC=C7)(CO4)OC(=O)C)O)C)O)OC(=O)C |
異性体SMILES |
CC1=C2[C@H]([C@@H]([C@@]3([C@H](C[C@@H]4[C@]([C@H]3[C@@H]([C@@](C2(C)C)(C[C@@H]1OC(=O)[C@@H]([C@H](C5=CC=CC=C5)NC(=O)C6=CC=CC=C6)O)O)OC(=O)C7=CC=CC=C7)(CO4)OC(=O)C)O)C)O)OC(=O)C |
正規SMILES |
CC1=C2C(C(C3(C(CC4C(C3C(C(C2(C)C)(CC1OC(=O)C(C(C5=CC=CC=C5)NC(=O)C6=CC=CC=C6)O)O)OC(=O)C7=CC=CC=C7)(CO4)OC(=O)C)O)C)O)OC(=O)C |
同義語 |
9(R)-dihydrotaxol 9-dihydro-taxol 9-dihydrotaxol |
製品の起源 |
United States |
試験管内研究製品の免責事項と情報
BenchChemで提示されるすべての記事および製品情報は、情報提供を目的としています。BenchChemで購入可能な製品は、生体外研究のために特別に設計されています。生体外研究は、ラテン語の "in glass" に由来し、生物体の外で行われる実験を指します。これらの製品は医薬品または薬として分類されておらず、FDAから任何の医療状態、病気、または疾患の予防、治療、または治癒のために承認されていません。これらの製品を人間または動物に体内に導入する形態は、法律により厳格に禁止されています。これらのガイドラインに従うことは、研究と実験において法的および倫理的な基準の遵守を確実にするために重要です。