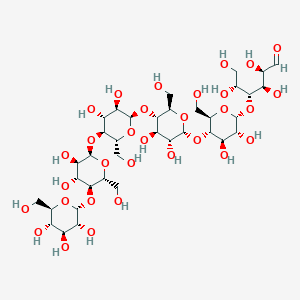
マルトヘキサオース
- 専門家チームからの見積もりを受け取るには、QUICK INQUIRYをクリックしてください。
- 品質商品を競争力のある価格で提供し、研究に集中できます。
説明
アミロヘキサオースは多糖類に分類され、アミロース、アミロペクチン、および全デンプンから誘導できます 。 この化合物はマルトデキストリンファミリーのメンバーであり、植物、特に大麦などの穀物に多く含まれています .
2. 製法
合成経路と反応条件: アミロヘキサオースは、特定のアミラーゼを用いたデンプンの酵素加水分解により合成できます。 このプロセスは、アミロースとアミロペクチンをアミロヘキサオースを含むより小さなオリゴ糖に分解することを伴います 。反応条件は通常、酵素活性を最適化するために、制御された温度とpHが含まれます。
工業生産方法: 工業的には、アミロヘキサオースは、微生物アミラーゼを用いたデンプンの酵素加水分解によって製造されます。 このプロセスは、デンプンをアミロヘキサオースに分解する能力を持つアミラーゼを生成する、エアロバクター・エアロゲネスなどのバクテリアの使用を伴います 。反応は、高収率と純度を確保するために、制御された条件下で大型バイオリアクターで行われます。
科学的研究の応用
アミロヘキサオースは、科学研究において幅広い用途を持っています。
作用機序
アミロヘキサオースは、主にアミラーゼなどの酵素との相互作用を通じて効果を発揮します。 アミロヘキサオース中のα-1,4-グリコシド結合はアミラーゼによって加水分解され、グルコース単位が放出されます 。このプロセスは、生体内の炭水化物の消化と利用に不可欠です。 分子標的は、グリコシド結合の加水分解が起こるアミラーゼの活性部位です .
類似の化合物:
セロヘキサオース: β-1,4-グリコシド結合によって結合した6つのグルコース単位で構成されています。
マンノヘキサオース: α-1,4-グリコシド結合によって結合した6つのマンノース単位で構成されています。
比較:
アミロヘキサオースとセロヘキサオース: アミロヘキサオースはα-1,4-グリコシド結合を持ち、らせん構造を形成しますが、セロヘキサオースはβ-1,4-グリコシド結合を持ち、直鎖状構造を形成します.
アミロヘキサオースとマンノヘキサオース: アミロヘキサオースはグルコース単位で構成されているのに対し、マンノヘキサオースはマンノース単位で構成されています。
アミロヘキサオースのユニークな構造と特性により、さまざまな科学的および産業的な用途において貴重な化合物となっています。
将来の方向性
Maltohexaose has shown promising preliminary results in rats infected with E. coli when used to differentiate live from dead bacteria in the early stages of the bacterial infection . It was both more sensitive and more specific than 18F-FDG, showing a 7-fold increase in tracer accumulation in infected tissue compared with the sterile .
生化学分析
Biochemical Properties
Maltohexaose interacts with various enzymes, proteins, and other biomolecules. For instance, it is the main product of the extracellular exo-maltohexaohydrolase enzyme from a Klebsiella pneumoniae mutant . This enzyme hydrolyzes short-chain amylose to produce about 40% maltohexaose . The enzyme also interacts with maltooligosaccharides larger than maltohexaose, producing mostly maltohexaose through an exo mechanism action .
Cellular Effects
It is known that maltohexaose is involved in the formation and hydrolysis of maltooligosaccharides . This suggests that maltohexaose may influence cell function, including impacts on cell signaling pathways, gene expression, and cellular metabolism.
Molecular Mechanism
Maltohexaose exerts its effects at the molecular level through various mechanisms. For instance, it is involved in the formation and hydrolysis of maltooligosaccharides by the exo-maltohexaohydrolase enzyme . This enzyme cleaves the α-1,4 glycosidic linkages of starch to produce maltohexaose . The enzyme’s action on maltohexaose is position-specific, with the highest frequency of cleavage at the second bond from the reducing end .
Temporal Effects in Laboratory Settings
It is known that maltohexaose is formed from maltotetraose by the transfer action of the exo-maltohexaohydrolase enzyme, with an action pattern dependent on the substrate concentration .
Metabolic Pathways
Maltohexaose is involved in the starch and sucrose metabolic pathway . It is produced from starch by the action of the exo-maltohexaohydrolase enzyme . This enzyme cleaves the α-1,4 glycosidic linkages of starch to produce maltohexaose .
Transport and Distribution
It is known that maltohexaose enters E.coli via the maltodextrin transport pathway .
Subcellular Localization
Given that it is a soluble sugar, it is likely to be found in the cytoplasm of cells
準備方法
Synthetic Routes and Reaction Conditions: Amylohexaose can be synthesized through the enzymatic hydrolysis of starch using specific amylases. The process involves the breakdown of amylose and amylopectin into smaller oligosaccharides, including amylohexaose . The reaction conditions typically include a controlled temperature and pH to optimize enzyme activity.
Industrial Production Methods: In industrial settings, amylohexaose is produced by enzymatic hydrolysis of starch using microbial amylases. The process involves the use of bacteria such as Aerobacter aerogenes, which produce amylases capable of breaking down starch into amylohexaose . The reaction is carried out in large bioreactors under controlled conditions to ensure high yield and purity.
化学反応の分析
反応の種類: アミロヘキサオースは、以下を含むさまざまな化学反応を起こします。
酸化: アミロヘキサオースは酸化してグルコン酸誘導体を生成できます。
還元: アミロヘキサオースの還元により、ソルビトールが生成されます。
置換: 置換反応により、アセチル基やリン酸基などの官能基が導入されます。
一般的な試薬と条件:
酸化: 一般的な酸化剤には、過酸化水素と過ヨウ素酸ナトリウムが含まれます。
還元: 水素化ホウ素ナトリウムなどの還元剤が使用されます。
置換: アセチル化またはリン酸化には、それぞれ無水酢酸またはリン酸を使用できます。
主要な生成物:
酸化: グルコン酸誘導体。
還元: ソルビトール。
置換: アセチル化またはリン酸化されたアミロヘキサオース。
類似化合物との比較
Cellohexaose: Composed of six glucose units linked by β-1,4-glycosidic bonds.
Mannohexaose: Composed of six mannose units linked by α-1,4-glycosidic bonds.
Comparison:
Amylohexaose vs. Cellohexaose: Amylohexaose has α-1,4-glycosidic bonds, resulting in a helical structure, whereas cellohexaose has β-1,4-glycosidic bonds, resulting in a linear structure.
Amylohexaose vs. Mannohexaose: Amylohexaose is composed of glucose units, while mannohexaose is composed of mannose units.
Amylohexaose’s unique structure and properties make it a valuable compound in various scientific and industrial applications.
特性
IUPAC Name |
2-[6-[6-[6-[4,5-dihydroxy-2-(hydroxymethyl)-6-[4,5,6-trihydroxy-2-(hydroxymethyl)oxan-3-yl]oxyoxan-3-yl]oxy-4,5-dihydroxy-2-(hydroxymethyl)oxan-3-yl]oxy-4,5-dihydroxy-2-(hydroxymethyl)oxan-3-yl]oxy-4,5-dihydroxy-2-(hydroxymethyl)oxan-3-yl]oxy-6-(hydroxymethyl)oxane-3,4,5-triol |
Source
|
---|---|---|
Details | Computed by Lexichem TK 2.7.0 (PubChem release 2021.05.07) | |
Source | PubChem | |
URL | https://pubchem.ncbi.nlm.nih.gov | |
Description | Data deposited in or computed by PubChem | |
InChI |
InChI=1S/C36H62O31/c37-1-7-13(43)14(44)21(51)32(58-7)64-27-9(3-39)60-34(23(53)16(27)46)66-29-11(5-41)62-36(25(55)18(29)48)67-30-12(6-42)61-35(24(54)19(30)49)65-28-10(4-40)59-33(22(52)17(28)47)63-26-8(2-38)57-31(56)20(50)15(26)45/h7-56H,1-6H2 |
Source
|
Details | Computed by InChI 1.0.6 (PubChem release 2021.05.07) | |
Source | PubChem | |
URL | https://pubchem.ncbi.nlm.nih.gov | |
Description | Data deposited in or computed by PubChem | |
InChI Key |
OCIBBXPLUVYKCH-UHFFFAOYSA-N |
Source
|
Details | Computed by InChI 1.0.6 (PubChem release 2021.05.07) | |
Source | PubChem | |
URL | https://pubchem.ncbi.nlm.nih.gov | |
Description | Data deposited in or computed by PubChem | |
Canonical SMILES |
C(C1C(C(C(C(O1)OC2C(OC(C(C2O)O)OC3C(OC(C(C3O)O)OC4C(OC(C(C4O)O)OC5C(OC(C(C5O)O)OC6C(OC(C(C6O)O)O)CO)CO)CO)CO)CO)O)O)O)O |
Source
|
Details | Computed by OEChem 2.3.0 (PubChem release 2021.05.07) | |
Source | PubChem | |
URL | https://pubchem.ncbi.nlm.nih.gov | |
Description | Data deposited in or computed by PubChem | |
Molecular Formula |
C36H62O31 |
Source
|
Details | Computed by PubChem 2.1 (PubChem release 2021.05.07) | |
Source | PubChem | |
URL | https://pubchem.ncbi.nlm.nih.gov | |
Description | Data deposited in or computed by PubChem | |
Molecular Weight |
990.9 g/mol |
Source
|
Details | Computed by PubChem 2.1 (PubChem release 2021.05.07) | |
Source | PubChem | |
URL | https://pubchem.ncbi.nlm.nih.gov | |
Description | Data deposited in or computed by PubChem | |
試験管内研究製品の免責事項と情報
BenchChemで提示されるすべての記事および製品情報は、情報提供を目的としています。BenchChemで購入可能な製品は、生体外研究のために特別に設計されています。生体外研究は、ラテン語の "in glass" に由来し、生物体の外で行われる実験を指します。これらの製品は医薬品または薬として分類されておらず、FDAから任何の医療状態、病気、または疾患の予防、治療、または治癒のために承認されていません。これらの製品を人間または動物に体内に導入する形態は、法律により厳格に禁止されています。これらのガイドラインに従うことは、研究と実験において法的および倫理的な基準の遵守を確実にするために重要です。