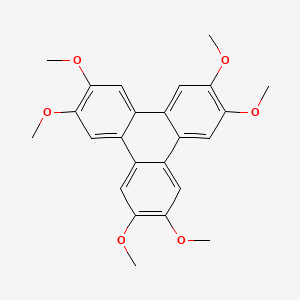
2,3,6,7,10,11-ヘキサメトキシトリフェニレン
概要
説明
2,3,6,7,10,11-Hexamethoxytriphenylene is an organic compound with the molecular formula C24H24O6 and a molecular weight of 408.44 g/mol . It is a fully conjugated triphenylene ligand, characterized by its planar disc-shaped structure and six methoxy groups at the ortho-positions . This compound is known for its high reversibility in electrochemical redox reactions, making it a valuable material in various scientific and industrial applications .
科学的研究の応用
2,3,6,7,10,11-Hexamethoxytriphenylene has a wide range of applications in scientific research, including:
生化学分析
Biochemical Properties
2,3,6,7,10,11-Hexamethoxytriphenylene plays a significant role in biochemical reactions, particularly in the context of its interactions with enzymes and proteins. It has been observed to interact with various biomolecules, influencing their activity and stability. For instance, the compound can act as a substrate or inhibitor for certain enzymes, thereby modulating their catalytic functions. The nature of these interactions is often dictated by the structural compatibility between 2,3,6,7,10,11-Hexamethoxytriphenylene and the active sites of the enzymes or proteins it interacts with .
Cellular Effects
The effects of 2,3,6,7,10,11-Hexamethoxytriphenylene on cellular processes are diverse and multifaceted. It has been shown to influence cell signaling pathways, gene expression, and cellular metabolism. For example, the compound can modulate the activity of key signaling molecules, leading to alterations in downstream cellular responses. Additionally, 2,3,6,7,10,11-Hexamethoxytriphenylene can affect the expression of specific genes, thereby impacting cellular functions such as proliferation, differentiation, and apoptosis .
Molecular Mechanism
At the molecular level, 2,3,6,7,10,11-Hexamethoxytriphenylene exerts its effects through various mechanisms. One of the primary modes of action involves binding interactions with biomolecules, such as enzymes and receptors. These interactions can lead to enzyme inhibition or activation, depending on the nature of the binding. Furthermore, 2,3,6,7,10,11-Hexamethoxytriphenylene can influence gene expression by interacting with transcription factors or other regulatory proteins, thereby modulating the transcriptional activity of target genes .
Temporal Effects in Laboratory Settings
In laboratory settings, the effects of 2,3,6,7,10,11-Hexamethoxytriphenylene can change over time. The compound’s stability and degradation are critical factors that influence its long-term effects on cellular function. Studies have shown that 2,3,6,7,10,11-Hexamethoxytriphenylene can undergo degradation under certain conditions, leading to the formation of metabolites with distinct biological activities. These temporal changes can impact the compound’s efficacy and safety in both in vitro and in vivo studies .
Dosage Effects in Animal Models
The effects of 2,3,6,7,10,11-Hexamethoxytriphenylene vary with different dosages in animal models. At lower doses, the compound may exhibit beneficial effects, such as enhancing cellular functions or protecting against oxidative stress. At higher doses, 2,3,6,7,10,11-Hexamethoxytriphenylene can induce toxic or adverse effects, including cellular damage and apoptosis. These dosage-dependent effects highlight the importance of determining the optimal therapeutic window for the compound’s use in biomedical applications .
Metabolic Pathways
2,3,6,7,10,11-Hexamethoxytriphenylene is involved in various metabolic pathways, interacting with enzymes and cofactors that facilitate its biotransformation. The compound can undergo phase I and phase II metabolic reactions, leading to the formation of metabolites that may possess distinct biological activities. These metabolic pathways can influence the compound’s pharmacokinetics and pharmacodynamics, affecting its overall efficacy and safety profile .
Transport and Distribution
The transport and distribution of 2,3,6,7,10,11-Hexamethoxytriphenylene within cells and tissues are critical determinants of its biological activity. The compound can interact with specific transporters or binding proteins that facilitate its uptake and distribution. Additionally, 2,3,6,7,10,11-Hexamethoxytriphenylene can accumulate in certain cellular compartments, influencing its localization and activity. Understanding these transport and distribution mechanisms is essential for optimizing the compound’s therapeutic potential .
Subcellular Localization
The subcellular localization of 2,3,6,7,10,11-Hexamethoxytriphenylene plays a crucial role in its activity and function. The compound can be directed to specific cellular compartments or organelles through targeting signals or post-translational modifications. This localization can impact the compound’s interactions with biomolecules and its overall biological effects. For instance, 2,3,6,7,10,11-Hexamethoxytriphenylene may localize to the nucleus, where it can influence gene expression, or to the mitochondria, where it can affect cellular metabolism .
準備方法
Synthetic Routes and Reaction Conditions
2,3,6,7,10,11-Hexamethoxytriphenylene can be synthesized through the oxidative trimerization of 1,2-dimethoxybenzene using transition metal compounds such as iron(III) chloride . The reaction typically involves the following steps:
Oxidative Trimerization: 1,2-dimethoxybenzene is oxidized using iron(III) chloride to form 2,3,6,7,10,11-hexamethoxytriphenylene.
Demethylation: The hexamethoxy compound can be further demethylated using strong Lewis acids like boron tribromide (BBr3) to yield hexahydroxytriphenylene.
Industrial Production Methods
The industrial production of 2,3,6,7,10,11-hexamethoxytriphenylene follows similar synthetic routes but on a larger scale. The process involves careful control of reaction conditions to ensure high yields and purity of the final product .
化学反応の分析
Types of Reactions
2,3,6,7,10,11-Hexamethoxytriphenylene undergoes various chemical reactions, including:
Oxidation: The compound can participate in electrochemical redox reactions, which are highly reversible.
Demethylation: Strong Lewis acids can demethylate the compound to form hexahydroxytriphenylene.
Common Reagents and Conditions
Oxidation: Iron(III) chloride is commonly used for the oxidative trimerization of 1,2-dimethoxybenzene.
Demethylation: Boron tribromide (BBr3) is used for the demethylation process.
Major Products Formed
Hexahydroxytriphenylene: Formed through the demethylation of 2,3,6,7,10,11-hexamethoxytriphenylene.
作用機序
The mechanism of action of 2,3,6,7,10,11-hexamethoxytriphenylene involves its ability to undergo reversible redox reactions. The compound’s planar structure and conjugated system facilitate efficient electron transfer, making it an effective material for applications in batteries and other electrochemical devices . The molecular targets and pathways involved include interactions with various metal ions and organic molecules, leading to the formation of stable complexes .
類似化合物との比較
2,3,6,7,10,11-Hexamethoxytriphenylene can be compared with other similar compounds, such as:
Triphenylene: The parent compound of 2,3,6,7,10,11-hexamethoxytriphenylene, lacking the methoxy groups.
Hexahydroxytriphenylene: The demethylated form of 2,3,6,7,10,11-hexamethoxytriphenylene, with hydroxyl groups instead of methoxy groups.
1,3,5-Tris(4-carboxyphenyl)benzene: Another triphenylene derivative used in the synthesis of COFs.
The uniqueness of 2,3,6,7,10,11-hexamethoxytriphenylene lies in its high reversibility in redox reactions and its ability to form stable complexes, making it a valuable material in various scientific and industrial applications .
特性
IUPAC Name |
2,3,6,7,10,11-hexamethoxytriphenylene | |
---|---|---|
Source | PubChem | |
URL | https://pubchem.ncbi.nlm.nih.gov | |
Description | Data deposited in or computed by PubChem | |
InChI |
InChI=1S/C24H24O6/c1-25-19-7-13-14(8-20(19)26-2)16-10-22(28-4)24(30-6)12-18(16)17-11-23(29-5)21(27-3)9-15(13)17/h7-12H,1-6H3 | |
Source | PubChem | |
URL | https://pubchem.ncbi.nlm.nih.gov | |
Description | Data deposited in or computed by PubChem | |
InChI Key |
TXROZCSFVVIBFI-UHFFFAOYSA-N | |
Source | PubChem | |
URL | https://pubchem.ncbi.nlm.nih.gov | |
Description | Data deposited in or computed by PubChem | |
Canonical SMILES |
COC1=C(C=C2C(=C1)C3=CC(=C(C=C3C4=CC(=C(C=C24)OC)OC)OC)OC)OC | |
Source | PubChem | |
URL | https://pubchem.ncbi.nlm.nih.gov | |
Description | Data deposited in or computed by PubChem | |
Molecular Formula |
C24H24O6 | |
Source | PubChem | |
URL | https://pubchem.ncbi.nlm.nih.gov | |
Description | Data deposited in or computed by PubChem | |
DSSTOX Substance ID |
DTXSID70404819 | |
Record name | 2,3,6,7,10,11-Hexamethoxytriphenylene | |
Source | EPA DSSTox | |
URL | https://comptox.epa.gov/dashboard/DTXSID70404819 | |
Description | DSSTox provides a high quality public chemistry resource for supporting improved predictive toxicology. | |
Molecular Weight |
408.4 g/mol | |
Source | PubChem | |
URL | https://pubchem.ncbi.nlm.nih.gov | |
Description | Data deposited in or computed by PubChem | |
CAS No. |
808-57-1 | |
Record name | 2,3,6,7,10,11-Hexamethoxytriphenylene | |
Source | EPA DSSTox | |
URL | https://comptox.epa.gov/dashboard/DTXSID70404819 | |
Description | DSSTox provides a high quality public chemistry resource for supporting improved predictive toxicology. | |
Synthesis routes and methods I
Procedure details
Synthesis routes and methods II
Procedure details
Synthesis routes and methods III
Procedure details
Synthesis routes and methods IV
Procedure details
Retrosynthesis Analysis
AI-Powered Synthesis Planning: Our tool employs the Template_relevance Pistachio, Template_relevance Bkms_metabolic, Template_relevance Pistachio_ringbreaker, Template_relevance Reaxys, Template_relevance Reaxys_biocatalysis model, leveraging a vast database of chemical reactions to predict feasible synthetic routes.
One-Step Synthesis Focus: Specifically designed for one-step synthesis, it provides concise and direct routes for your target compounds, streamlining the synthesis process.
Accurate Predictions: Utilizing the extensive PISTACHIO, BKMS_METABOLIC, PISTACHIO_RINGBREAKER, REAXYS, REAXYS_BIOCATALYSIS database, our tool offers high-accuracy predictions, reflecting the latest in chemical research and data.
Strategy Settings
Precursor scoring | Relevance Heuristic |
---|---|
Min. plausibility | 0.01 |
Model | Template_relevance |
Template Set | Pistachio/Bkms_metabolic/Pistachio_ringbreaker/Reaxys/Reaxys_biocatalysis |
Top-N result to add to graph | 6 |
Feasible Synthetic Routes
Q1: What is the molecular structure and formula of 2,3,6,7,10,11-Hexamethoxytriphenylene?
A1: 2,3,6,7,10,11-Hexamethoxytriphenylene (HMTP) comprises a central triphenylene core, a polyaromatic hydrocarbon, decorated with six methoxy (-OCH3) groups. Its molecular formula is C24H24O6. [, , , ]
Q2: How does HMTP interact with surfaces like silver (Ag(111)) and graphene?
A2: HMTP exhibits weak interactions with graphene, forming a commensurate close-packed hexagonal network on graphene/Ir(111). [] Conversely, on Ag(111) surfaces, HMTP interacts weakly while its acceptor counterpart, HATCN, demonstrates strong interactions, leading to charge transfer and surface buckling. Interestingly, mixing HMTP and HATCN results in strong hybridization and the emergence of a common unoccupied molecular orbital, signifying potential for charge-transfer complex formation. []
Q3: Can HMTP be used as a component in organic electronic devices?
A3: Research suggests that HMTP, as an electron donor, can form charge-transfer complexes with acceptor molecules like HATCN. [] These complexes are crucial for developing organic electronic devices like organic solar cells and transistors.
Q4: Are there alternative methods to synthesize HMTP besides traditional chemical routes?
A4: Yes, electrochemical methods offer a greener approach to synthesizing HMTP derivatives. Anodic oxidation of catechol ketals, followed by acidic hydrolysis, can yield high-quality HMTP. This method minimizes metal waste and avoids harsh reaction conditions. []
Q5: What is known about the crystal structure of HMTP?
A5: HMTP crystallizes differently than expected for typical discotic columnar mesogens. Instead of stacking based on the aromatic core, its crystal structure is primarily governed by the arrangement of alkyl groups. [] This insight highlights the importance of peripheral groups in influencing the self-assembly and ultimately the material properties of HMTP.
Q6: What are the challenges associated with studying HMTP adsorption on alkali halide surfaces?
A7: Studying molecular adsorption on bulk insulator surfaces like KBr(001) is challenging due to the limited number of molecules suitable for such investigations. HMTP, with its flat aromatic core and peripheral polar groups, was specifically designed to enhance interactions with KBr(001) and minimize molecular diffusion at room temperature. []
Q7: How does the structure of HMTP contribute to its properties and potential applications?
A8: The planar aromatic core of HMTP facilitates π-π stacking interactions, which are crucial for charge transport in organic electronics. [] Additionally, the six methoxy groups enhance solubility and influence the molecule's self-assembly behavior, impacting its potential applications in various fields. []
試験管内研究製品の免責事項と情報
BenchChemで提示されるすべての記事および製品情報は、情報提供を目的としています。BenchChemで購入可能な製品は、生体外研究のために特別に設計されています。生体外研究は、ラテン語の "in glass" に由来し、生物体の外で行われる実験を指します。これらの製品は医薬品または薬として分類されておらず、FDAから任何の医療状態、病気、または疾患の予防、治療、または治癒のために承認されていません。これらの製品を人間または動物に体内に導入する形態は、法律により厳格に禁止されています。これらのガイドラインに従うことは、研究と実験において法的および倫理的な基準の遵守を確実にするために重要です。