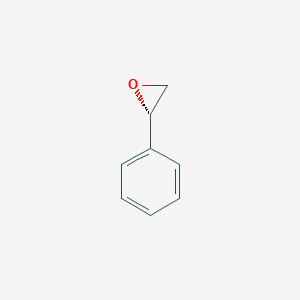
(R)-スチレンオキシド
概要
説明
(R)-styrene oxide is the (R)-enantiomer of styrene oxide. It is an enantiomer of a (S)-styrene oxide.
科学的研究の応用
抵抗変化型メモリデバイス
(R)-スチレンオキシドは、抵抗変化型ランダムアクセスメモリ(RRAM)などの抵抗変化型メモリデバイスの製造における潜在的な用途について調査されています . これらのデバイスは、誘電体固体材料の抵抗を変化させることによって動作する、不揮発性記憶の一種です。 (R)-スチレンオキシドは、金属酸化物の表面特性を修飾するために使用でき、メモリコンピューティングおよび情報記憶用途における性能を向上させることができます。
ナノ粒子合成
この化合物は、さまざまなナノテクノロジー用途で重要な役割を果たす金属酸化物ナノ粒子の合成において役割を果たします . (R)-スチレンオキシドは、ナノ粒子の表面修飾に関係しており、他の物質との相互作用を改善し、これは高感度で選択的なセンサーを作成するために不可欠です。
触媒
触媒において、(R)-スチレンオキシドは、収率と選択性を向上させた触媒の製造に使用されます . 分子状酸素を唯一の酸化剤として、シクロペンテンからシクロペンタノンを製造する触媒系の一部となることができます。 この用途は、高い転換率と選択性が求められる産業プロセスで重要です。
ドラッグデリバリーシステム
この化合物の反応性により、ドラッグデリバリーシステムで使用される官能基化シリカナノ粒子の作成が可能になります . (R)-スチレンオキシドは、シリカベースのナノ粒子の表面を修飾するために使用でき、標的療法の重要な側面である、制御された方法で薬物を運搬および放出する能力を向上させることができます。
環境修復
(R)-スチレンオキシドは、環境修復技術で使用される可能性があります。 その化学的特性は、水や空気から汚染物質を吸着および除去するために使用される材料の開発に役立ちます . この用途は、特に産業排水の浄化と、よりクリーンな環境慣行を確保するために重要です。
エネルギー貯蔵
この化合物は、エネルギー貯蔵の分野でも関連しています。 リチウムイオン電池やスーパーキャパシタの材料開発に使用でき、エネルギー密度と効率の向上に貢献します . これは、再生可能エネルギー技術を進歩させ、より持続可能なエネルギー貯蔵ソリューションを作成するために不可欠です。
作用機序
Target of Action
It is known that similar compounds interact with various molecular targets, including reactive oxygen species (ros) and reactive nitrogen species (rns) . These molecules play crucial roles in various physiological functions such as immune function, cellular signaling pathways, and redox regulation .
Mode of Action
It can be inferred from related studies that such compounds may interact with their targets through redox reactions . For instance, nitric oxide, a free radical, has been shown to interact with other molecules by donating or accepting an electron .
Biochemical Pathways
It is known that ros/rns, which could be potential targets of ®-styrene oxide, are involved in various biochemical transformations such as carboxylation, hydroxylation, peroxidation, and modulation of signal transduction pathways .
Pharmacokinetics
It is known that the adme properties of a compound significantly influence its bioavailability and efficacy .
Result of Action
It is known that ros/rns, potential targets of ®-styrene oxide, can cause damage to cellular biomolecules, including lipids, proteins, and dna, leading to various chronic and degenerative diseases .
Action Environment
Environmental factors can significantly influence the action, efficacy, and stability of a compound. For instance, pollution, radiation, certain drugs, foods, cigarette smoking, and other exogenous sources can increase the levels of ROS/RNS, thereby potentially influencing the action of ®-Styrene oxide .
特性
IUPAC Name |
(2R)-2-phenyloxirane | |
---|---|---|
Source | PubChem | |
URL | https://pubchem.ncbi.nlm.nih.gov | |
Description | Data deposited in or computed by PubChem | |
InChI |
InChI=1S/C8H8O/c1-2-4-7(5-3-1)8-6-9-8/h1-5,8H,6H2/t8-/m0/s1 | |
Source | PubChem | |
URL | https://pubchem.ncbi.nlm.nih.gov | |
Description | Data deposited in or computed by PubChem | |
InChI Key |
AWMVMTVKBNGEAK-QMMMGPOBSA-N | |
Source | PubChem | |
URL | https://pubchem.ncbi.nlm.nih.gov | |
Description | Data deposited in or computed by PubChem | |
Canonical SMILES |
C1C(O1)C2=CC=CC=C2 | |
Source | PubChem | |
URL | https://pubchem.ncbi.nlm.nih.gov | |
Description | Data deposited in or computed by PubChem | |
Isomeric SMILES |
C1[C@H](O1)C2=CC=CC=C2 | |
Source | PubChem | |
URL | https://pubchem.ncbi.nlm.nih.gov | |
Description | Data deposited in or computed by PubChem | |
Molecular Formula |
C8H8O | |
Source | PubChem | |
URL | https://pubchem.ncbi.nlm.nih.gov | |
Description | Data deposited in or computed by PubChem | |
Molecular Weight |
120.15 g/mol | |
Source | PubChem | |
URL | https://pubchem.ncbi.nlm.nih.gov | |
Description | Data deposited in or computed by PubChem | |
CAS No. |
20780-53-4 | |
Record name | (+)-Styrene oxide | |
Source | CAS Common Chemistry | |
URL | https://commonchemistry.cas.org/detail?cas_rn=20780-53-4 | |
Description | CAS Common Chemistry is an open community resource for accessing chemical information. Nearly 500,000 chemical substances from CAS REGISTRY cover areas of community interest, including common and frequently regulated chemicals, and those relevant to high school and undergraduate chemistry classes. This chemical information, curated by our expert scientists, is provided in alignment with our mission as a division of the American Chemical Society. | |
Explanation | The data from CAS Common Chemistry is provided under a CC-BY-NC 4.0 license, unless otherwise stated. | |
Record name | Styrene oxide, (R)- | |
Source | ChemIDplus | |
URL | https://pubchem.ncbi.nlm.nih.gov/substance/?source=chemidplus&sourceid=0020780534 | |
Description | ChemIDplus is a free, web search system that provides access to the structure and nomenclature authority files used for the identification of chemical substances cited in National Library of Medicine (NLM) databases, including the TOXNET system. | |
Record name | (R)-styrene oxide | |
Source | DrugBank | |
URL | https://www.drugbank.ca/drugs/DB04499 | |
Description | The DrugBank database is a unique bioinformatics and cheminformatics resource that combines detailed drug (i.e. chemical, pharmacological and pharmaceutical) data with comprehensive drug target (i.e. sequence, structure, and pathway) information. | |
Explanation | Creative Common's Attribution-NonCommercial 4.0 International License (http://creativecommons.org/licenses/by-nc/4.0/legalcode) | |
Record name | STYRENE OXIDE, (R)- | |
Source | FDA Global Substance Registration System (GSRS) | |
URL | https://gsrs.ncats.nih.gov/ginas/app/beta/substances/0HB27D5MRW | |
Description | The FDA Global Substance Registration System (GSRS) enables the efficient and accurate exchange of information on what substances are in regulated products. Instead of relying on names, which vary across regulatory domains, countries, and regions, the GSRS knowledge base makes it possible for substances to be defined by standardized, scientific descriptions. | |
Explanation | Unless otherwise noted, the contents of the FDA website (www.fda.gov), both text and graphics, are not copyrighted. They are in the public domain and may be republished, reprinted and otherwise used freely by anyone without the need to obtain permission from FDA. Credit to the U.S. Food and Drug Administration as the source is appreciated but not required. | |
Synthesis routes and methods I
Procedure details
Synthesis routes and methods II
Procedure details
Synthesis routes and methods III
Procedure details
Synthesis routes and methods IV
Procedure details
Retrosynthesis Analysis
AI-Powered Synthesis Planning: Our tool employs the Template_relevance Pistachio, Template_relevance Bkms_metabolic, Template_relevance Pistachio_ringbreaker, Template_relevance Reaxys, Template_relevance Reaxys_biocatalysis model, leveraging a vast database of chemical reactions to predict feasible synthetic routes.
One-Step Synthesis Focus: Specifically designed for one-step synthesis, it provides concise and direct routes for your target compounds, streamlining the synthesis process.
Accurate Predictions: Utilizing the extensive PISTACHIO, BKMS_METABOLIC, PISTACHIO_RINGBREAKER, REAXYS, REAXYS_BIOCATALYSIS database, our tool offers high-accuracy predictions, reflecting the latest in chemical research and data.
Strategy Settings
Precursor scoring | Relevance Heuristic |
---|---|
Min. plausibility | 0.01 |
Model | Template_relevance |
Template Set | Pistachio/Bkms_metabolic/Pistachio_ringbreaker/Reaxys/Reaxys_biocatalysis |
Top-N result to add to graph | 6 |
Feasible Synthetic Routes
Q1: What is the molecular formula and weight of (R)-styrene oxide?
A1: (R)-Styrene oxide has the molecular formula C8H8O and a molecular weight of 120.15 g/mol.
Q2: Are there any characteristic spectroscopic data for identifying (R)-styrene oxide?
A2: Yes, (R)-styrene oxide can be identified using techniques like NMR spectroscopy. For instance, researchers utilized 1H NMR analysis of the bis-(+)-α-(methoxy-α-trifluoromethyl)phenylacetate diesters to determine the stereochemical course of styrene oxide hydrolysis. []
Q3: Is (R)-styrene oxide stable under standard storage conditions?
A3: While specific data on the stability of (R)-styrene oxide under standard conditions wasn't found within the provided research, it's known that epoxides, in general, are susceptible to ring-opening reactions under various conditions, including acidic or basic environments.
Q4: What types of reactions can (R)-styrene oxide undergo?
A4: (R)-Styrene oxide is commonly used as a chiral building block in organic synthesis. It can undergo various reactions, including ring-opening reactions with nucleophiles such as alcohols, amines, and thiols. For example, researchers used (R)-styrene oxide as a starting material for the synthesis of chiral diamines via aziridinium ion intermediates. []
Q5: Can you give specific examples of catalysts used for (R)-styrene oxide reactions?
A5: Several catalysts have been reported for (R)-styrene oxide transformations. These include:
- Lewis Acids: Bis-tributyltin oxide and bis-chlorodibutyltin oxide for regio- and stereoselective alcoholysis []
- Magnesium Oxide: Catalyzes the cycloaddition of carbon dioxide to (R)-styrene oxide to yield (R)-phenyl carbonate with retention of stereochemistry. []
- Cerium Triflate [Ce(OTf)4]: Enables highly efficient, regio-, and stereoselective ring opening of (R)-styrene oxide with various nucleophiles like alcohols. []
Q6: What is the significance of enantioselective hydrolysis of (R)-styrene oxide?
A6: Enantioselective hydrolysis of (R)-styrene oxide, often catalyzed by enzymes like epoxide hydrolases, is crucial for producing enantiomerically pure compounds. For example, researchers used a recombinant E. coli expressing epoxide hydrolase for the kinetic resolution of racemic styrene oxide, achieving enantiopure (R)-styrene oxide. []
Q7: Has computational chemistry been used in research related to (R)-styrene oxide?
A7: Yes, computational chemistry plays a role in understanding the interactions of (R)-styrene oxide with enzymes. For example, docking simulations were used to study the binding of (R)-styrene oxide in the active site of cytochrome P450SPα, providing insights into the stereoselective epoxidation process. []
Q8: What are the known toxicological effects of (R)-styrene oxide?
A8: (R)-styrene oxide is known to be more toxic than its (S)-enantiomer. Studies in mice showed that (R)-styrene oxide caused greater toxicity, especially in the liver, as measured by serum sorbitol dehydrogenase (SDH) activity. []
Q9: What role does glutathione play in the toxicity of styrene and its metabolites?
A9: Glutathione (GSH) is a crucial antioxidant. Studies showed that styrene administration to mice led to a significant decrease in GSH levels in both bronchoalveolar lavage fluid (BALF) and plasma. This depletion of GSH, particularly in the lung, might contribute to the susceptibility to oxidative damage caused by styrene and its metabolites. []
Q10: Are there specific biomarkers associated with styrene or (R)-styrene oxide exposure?
A10: Researchers used elevated levels of gamma-glutamyltranspeptidase (GGT) and lactate dehydrogenase (LDH) in bronchoalveolar lavage fluid (BALF) as biomarkers for assessing pneumotoxicity caused by styrene and styrene oxide. []
Q11: How is (R)-styrene oxide typically analyzed and quantified?
A11: Various analytical methods are employed for analyzing (R)-styrene oxide, including:
- Gas Chromatography (GC): Used to determine enantiomeric excess and monitor the kinetic resolution of styrene oxide. [, ]
- High-Performance Liquid Chromatography (HPLC): Used for separating and quantifying (R)-styrene oxide in mixtures. []
- NMR Spectroscopy: Employed for stereochemical analysis of reaction products involving (R)-styrene oxide. []
Q12: Are there specialized resources available for research on (R)-styrene oxide?
A12: While specific databases dedicated solely to (R)-styrene oxide might not exist, resources such as:
Q13: What are some key milestones in the research on (R)-styrene oxide?
A13: * Discovery of Stereoselective Metabolism: A significant milestone was the understanding that the metabolism of styrene to styrene oxide is stereoselective, leading to different ratios of (R)- and (S)-styrene oxide in various species. []* Development of Biocatalytic Processes: Research on enzymes like epoxide hydrolases led to the development of biocatalytic processes for the enantioselective resolution of racemic styrene oxide. [, ]
試験管内研究製品の免責事項と情報
BenchChemで提示されるすべての記事および製品情報は、情報提供を目的としています。BenchChemで購入可能な製品は、生体外研究のために特別に設計されています。生体外研究は、ラテン語の "in glass" に由来し、生物体の外で行われる実験を指します。これらの製品は医薬品または薬として分類されておらず、FDAから任何の医療状態、病気、または疾患の予防、治療、または治癒のために承認されていません。これらの製品を人間または動物に体内に導入する形態は、法律により厳格に禁止されています。これらのガイドラインに従うことは、研究と実験において法的および倫理的な基準の遵守を確実にするために重要です。