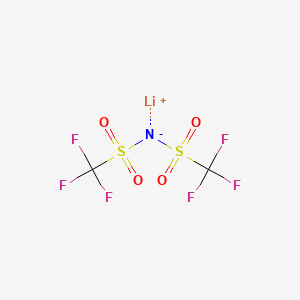
ビス(トリフルオロメタンスルホニル)イミドリチウム
概要
説明
Bis(trifluoromethane)sulfonimide lithium salt is a synthetic reagent.
科学的研究の応用
有機太陽電池
Li-TFSIは、化学添加剤として使用され、ポルフィリン系有機太陽電池の電力変換効率を向上させることができます .
重合イオン液体
これは、イミダゾリウムコアを有するモノマーイオン液体の調製における試薬として機能し、続いて重合イオン液体を開発するために使用されます .
有機電解質ベースのリチウム電池
Li-TFSIは、有機電解質ベースのリチウム電池の調製に使用され、高性能エネルギー貯蔵システムの開発に貢献しています .
キラルイミダゾリウム塩
この化合物は、アニオン交換プロセスを通じてキラルイミダゾリウム塩の合成に関与しており、不斉合成と触媒作用に影響を与えます .
希土類ルイス酸触媒
Li-TFSIは、さまざまな化学反応およびプロセスにとって重要な希土類ルイス酸触媒の調製において応用が見られます .
リチウムイオン電池用液体電解質
これは、カソードとアノード間のリチウムイオンの伝導を可能にする液体電解質の一部として、商業用リチウムイオン電池において重要な役割を果たします .
生化学分析
Biochemical Properties
Lithium bis((trifluoromethyl)sulfonyl)azanide plays a significant role in biochemical reactions, particularly in the context of its interactions with enzymes, proteins, and other biomolecules. The compound’s anion, bistriflimide, coordinates weakly with cations, which can influence the activity of various enzymes and proteins. For instance, it has been shown to interact with carbonyl groups in certain covalent organic frameworks, forming hydrogen bonds that can affect the transfer number of lithium ions . These interactions can modulate the activity of enzymes involved in metabolic pathways, thereby influencing biochemical reactions.
Cellular Effects
Lithium bis((trifluoromethyl)sulfonyl)azanide has notable effects on various types of cells and cellular processes. It influences cell function by affecting cell signaling pathways, gene expression, and cellular metabolism. The compound’s high solubility and ability to coordinate weakly with cations allow it to penetrate cell membranes and interact with intracellular components. This can lead to alterations in cell signaling pathways, such as those involving calcium ions, and changes in gene expression that impact cellular metabolism .
Molecular Mechanism
The molecular mechanism of action of lithium bis((trifluoromethyl)sulfonyl)azanide involves its interactions with biomolecules at the molecular level. The compound’s anion, bistriflimide, coordinates weakly with cations, which can lead to enzyme inhibition or activation. For example, it can inhibit enzymes involved in the degradation of certain metabolites, thereby altering metabolic flux. Additionally, lithium bis((trifluoromethyl)sulfonyl)azanide can bind to specific proteins, leading to changes in their conformation and activity .
Temporal Effects in Laboratory Settings
In laboratory settings, the effects of lithium bis((trifluoromethyl)sulfonyl)azanide can change over time. The compound’s stability and degradation are critical factors that influence its long-term effects on cellular function. Studies have shown that lithium bis((trifluoromethyl)sulfonyl)azanide can cause damage to the nervous system through prolonged or repeated exposure . Additionally, its high solubility in water ensures that it remains active in aqueous environments, maintaining its biochemical effects over extended periods.
Dosage Effects in Animal Models
The effects of lithium bis((trifluoromethyl)sulfonyl)azanide vary with different dosages in animal models. At low doses, the compound can enhance the activity of certain enzymes and proteins, leading to beneficial effects on cellular function. At high doses, lithium bis((trifluoromethyl)sulfonyl)azanide can cause toxic or adverse effects, such as severe skin burns and eye damage . Threshold effects have been observed, indicating that there is a specific dosage range within which the compound exerts its optimal effects without causing toxicity.
Metabolic Pathways
Lithium bis((trifluoromethyl)sulfonyl)azanide is involved in various metabolic pathways, interacting with enzymes and cofactors that regulate metabolic flux. The compound’s anion, bistriflimide, can inhibit enzymes involved in the degradation of certain metabolites, leading to changes in metabolite levels. Additionally, lithium bis((trifluoromethyl)sulfonyl)azanide can affect the activity of enzymes that regulate the synthesis and breakdown of key biomolecules, thereby influencing overall metabolic pathways .
Transport and Distribution
Within cells and tissues, lithium bis((trifluoromethyl)sulfonyl)azanide is transported and distributed through interactions with transporters and binding proteins. The compound’s high solubility in water facilitates its movement across cell membranes and its accumulation in specific cellular compartments. This can lead to localized effects on cellular function, depending on the concentration and distribution of lithium bis((trifluoromethyl)sulfonyl)azanide within the cell .
Subcellular Localization
The subcellular localization of lithium bis((trifluoromethyl)sulfonyl)azanide is influenced by targeting signals and post-translational modifications that direct it to specific compartments or organelles. The compound can accumulate in organelles such as mitochondria and lysosomes, where it can exert its effects on cellular metabolism and function. This localization is critical for its activity, as it allows lithium bis((trifluoromethyl)sulfonyl)azanide to interact with specific biomolecules and modulate their activity .
特性
CAS番号 |
90076-65-6 |
---|---|
分子式 |
C2HF6LiNO4S2 |
分子量 |
288.1 g/mol |
IUPAC名 |
lithium;bis(trifluoromethylsulfonyl)azanide |
InChI |
InChI=1S/C2HF6NO4S2.Li/c3-1(4,5)14(10,11)9-15(12,13)2(6,7)8;/h9H; |
InChIキー |
UGEOMRBXRFUYJH-UHFFFAOYSA-N |
SMILES |
[Li+].C(F)(F)(F)S(=O)(=O)[N-]S(=O)(=O)C(F)(F)F |
正規SMILES |
[Li].C(F)(F)(F)S(=O)(=O)NS(=O)(=O)C(F)(F)F |
90076-65-6 | |
物理的記述 |
PelletsLargeCrystals |
ピクトグラム |
Corrosive; Acute Toxic; Health Hazard |
製品の起源 |
United States |
Synthesis routes and methods
Procedure details
Retrosynthesis Analysis
AI-Powered Synthesis Planning: Our tool employs the Template_relevance Pistachio, Template_relevance Bkms_metabolic, Template_relevance Pistachio_ringbreaker, Template_relevance Reaxys, Template_relevance Reaxys_biocatalysis model, leveraging a vast database of chemical reactions to predict feasible synthetic routes.
One-Step Synthesis Focus: Specifically designed for one-step synthesis, it provides concise and direct routes for your target compounds, streamlining the synthesis process.
Accurate Predictions: Utilizing the extensive PISTACHIO, BKMS_METABOLIC, PISTACHIO_RINGBREAKER, REAXYS, REAXYS_BIOCATALYSIS database, our tool offers high-accuracy predictions, reflecting the latest in chemical research and data.
Strategy Settings
Precursor scoring | Relevance Heuristic |
---|---|
Min. plausibility | 0.01 |
Model | Template_relevance |
Template Set | Pistachio/Bkms_metabolic/Pistachio_ringbreaker/Reaxys/Reaxys_biocatalysis |
Top-N result to add to graph | 6 |
Feasible Synthetic Routes
Q1: Why is LiTFSI considered a promising electrolyte salt for lithium-ion batteries?
A1: LiTFSI exhibits several advantageous properties over conventional lithium hexafluorophosphate (LiPF6), including:
- Higher thermal stability: This leads to improved safety and performance at elevated temperatures. [, ]
- Resistance to hydrolysis: This enhances moisture resistance, simplifying battery manufacturing and extending shelf life. []
- High ionic conductivity: LiTFSI-based electrolytes facilitate efficient ion transport, enabling high-rate performance. [, , , ]
Q2: How does LiTFSI interact with electrode materials like silicon and impact battery performance?
A3: In silicon anodes, the interaction between LiTFSI and the silicon surface can lead to the formation of an unstable solid electrolyte interphase (SEI), resulting in capacity fading. Researchers are exploring strategies like incorporating electrolyte additives and novel binders to mitigate these issues. [, ]
Q3: What strategies can mitigate aluminum corrosion caused by LiTFSI in high-voltage batteries?
A3: While LiTFSI offers benefits, it can corrode aluminum current collectors at high voltages. Strategies to circumvent this include:
- Low-voltage operation: Limiting the cell voltage below the threshold for aluminum corrosion. []
- Salt additives: Incorporating additives like lithium tetrafluoroborate (LiBF4) or lithium difluoro(oxalato)borate (LiDFOB) to suppress corrosion. []
Q4: What is the role of LiTFSI in gel polymer electrolytes (GPEs)?
A6: In GPEs, LiTFSI serves as the primary source of charge carriers. It dissolves in a polymer matrix and dissociates into Li+ and TFSI- ions, enabling ionic conductivity. The properties of the GPE, such as ionic conductivity and electrochemical stability, depend on the polymer-salt interactions and the concentration of LiTFSI. []
Q5: How does the presence of LiTFSI affect the polymerization of methyl methacrylate (MMA)?
A7: LiTFSI, typically considered a poor initiator for MMA polymerization, can be used in conjunction with fluoroethylene carbonate (FEC). FEC's high dielectric constant promotes LiTFSI dissociation, enhancing its ability to initiate MMA polymerization. This approach facilitates the creation of novel polymer electrolytes with desirable properties for lithium metal batteries. []
Q6: What is the molecular formula and weight of LiTFSI?
A6: The molecular formula of LiTFSI is C2F6LiNO4S2, and its molecular weight is 287.09 g/mol.
Q7: What spectroscopic techniques are commonly employed to characterize LiTFSI?
A7: Common techniques include:
- Fourier-transform infrared spectroscopy (FTIR): This method helps identify functional groups and analyze interactions between LiTFSI and other components in electrolytes. []
- Nuclear magnetic resonance (NMR) spectroscopy: NMR provides insights into the solvation structure and dynamics of LiTFSI in various solvents. []
- X-ray photoelectron spectroscopy (XPS): This surface-sensitive technique is valuable for analyzing the composition and chemical states of SEI layers formed in the presence of LiTFSI. [, ]
Q8: What is the role of additives like fluoroethylene carbonate (FEC) in LiTFSI-based electrolytes?
A11: FEC acts as a solid electrolyte interphase (SEI)-forming additive, contributing to a more stable and robust SEI layer on electrode surfaces. This, in turn, improves the cycling performance and lifespan of the battery. []
Q9: How does the thermal stability of LiTFSI contribute to battery safety?
A13: LiTFSI's higher thermal stability compared to LiPF6 reduces the risk of electrolyte decomposition and thermal runaway at elevated temperatures, contributing to safer battery operation. [, ]
Q10: Are there any environmental concerns associated with LiTFSI?
A14: While LiTFSI offers advantages over LiPF6, its potential environmental impact requires further investigation. Research on its biodegradability, ecotoxicological effects, and sustainable disposal methods is crucial. []
Q11: How is computational chemistry employed in LiTFSI research?
A11: Computational methods such as molecular dynamics (MD) simulations and density functional theory (DFT) calculations are essential for:
- Understanding ion transport mechanisms: Simulations provide insights into the movement of Li+ and TFSI- ions in different electrolyte systems. [, , ]
- Predicting electrolyte properties: Computational models help predict ionic conductivity, viscosity, and other critical parameters based on electrolyte composition. []
- Designing novel electrolyte materials: Simulations guide the development of new LiTFSI-based electrolytes with improved performance and safety characteristics. [, ]
試験管内研究製品の免責事項と情報
BenchChemで提示されるすべての記事および製品情報は、情報提供を目的としています。BenchChemで購入可能な製品は、生体外研究のために特別に設計されています。生体外研究は、ラテン語の "in glass" に由来し、生物体の外で行われる実験を指します。これらの製品は医薬品または薬として分類されておらず、FDAから任何の医療状態、病気、または疾患の予防、治療、または治癒のために承認されていません。これらの製品を人間または動物に体内に導入する形態は、法律により厳格に禁止されています。これらのガイドラインに従うことは、研究と実験において法的および倫理的な基準の遵守を確実にするために重要です。