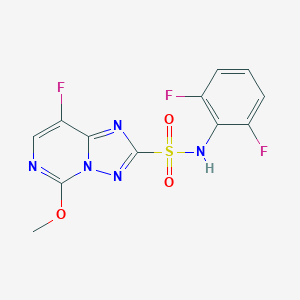
フロラスラム
概要
説明
Florasulam is a herbicide used to control broadleaf weeds in a variety of crops. It is a member of the sulfonylurea family of herbicides, and is one of the most widely used active ingredients in herbicides. Florasulam works by inhibiting the growth of weeds, and is known to be effective against a variety of broadleaf weeds, including dandelions, pigweed, and henbit.
科学的研究の応用
水生環境における光分解
フロラスラムは、UV-可視光照射下での水における光分解特性について研究されています。 研究では、光源の種類、濃度、水源、pHレベルなどのさまざまな要因を考慮したフロラスラムの分解速度論が調査されています . 硝酸イオンや特定の金属の存在は、分解速度に影響を与える可能性があり、水生システムにおけるフロラスラムの環境影響を理解するために重要です .
除草剤メカニズム分析
トリアゾロピリミジンスルホンアニリド系除草剤であるフロラスラムは、スルホニルウレア系除草剤と同様の除草作用を示します。 それはアセト乳酸シンターゼ(ALS)阻害剤として機能し、そのメカニズムは、農業におけるその有効性と選択性を高めるために広く研究されてきました .
変換生成物の同定
光分解中のフロラスラムの変換生成物(TP)の同定は、環境モニタリングにとって重要です。 UPLC-QTOF-MSなどの高度な技術を使用して、TPを分離および同定し、分解経路と安定なTPの潜在的な環境リスクを理解してきました .
環境安全評価
フロラスラムは、特に土壌や水における環境への影響について評価されています。 土壌における難分解性と他の除草剤との混和性は、環境安全評価の対象となっています .
芝生管理
芝生管理では、フロラスラムを含む製剤が、広葉雑草の防除に使用されています。 その選択性と環境安全性、費用対効果は、高品質の芝生表面を維持するための貴重なツールとなっています .
農薬リスク評価
フロラスラムは、冬小麦や春麦、トウモロコシ、牧草などのさまざまな作物における除草剤としての使用について、農薬リスク評価が行われています。 これらの評価は、農業におけるフロラスラムの安全な使用を確保するために不可欠です .
作用機序
Target of Action
Florasulam is a selective herbicide that primarily targets a plant’s enzyme system, specifically the acetolactate synthase (ALS) enzyme . This enzyme plays a crucial role in the synthesis of essential amino acids that are vital for plant growth .
Mode of Action
Florasulam operates by inhibiting the growth of weeds through the disruption of the plant’s enzyme system . By inhibiting the ALS enzyme, florasulam effectively stops weed growth, leading to their eventual death . Importantly, the turf grass, being less sensitive to this mode of action, remains unaffected and healthy .
Biochemical Pathways
The primary biochemical pathway affected by florasulam is the synthesis of essential amino acids in plants . By inhibiting the ALS enzyme, florasulam disrupts this pathway, effectively halting the growth of the plant . The downstream effects of this disruption include the cessation of plant growth and eventual death of the plant .
Pharmacokinetics
It is known that florasulam is highly soluble in water , which can influence its bioavailability and distribution in the environment .
Result of Action
The result of florasulam’s action is the effective control of broad-leaved weeds without damaging the turf grass . By inhibiting the ALS enzyme, florasulam stops weed growth, leading to their eventual death . This selective action makes florasulam an excellent choice for maintaining the aesthetics and health of lawns .
Action Environment
Florasulam is highly soluble in water and volatile, with a possibility of leaching to groundwater under certain conditions . Environmental factors such as soil type, rainfall, and temperature can influence the action, efficacy, and stability of florasulam . Proper adherence to application guidelines is crucial to ensure the safety of the environment .
将来の方向性
生化学分析
Biochemical Properties
Florasulam interacts with various enzymes and proteins in biochemical reactions. It is a typical acetolactate synthase (ALS) inhibitor . ALS is a key enzyme in the biosynthesis of branched-chain amino acids. By inhibiting this enzyme, Florasulam prevents the synthesis of these amino acids, thereby inhibiting the growth of broad-leaved weeds .
Cellular Effects
Florasulam has significant effects on various types of cells and cellular processes. After exposure to Florasulam, reactive oxygen species levels in larvae and adult zebrafish significantly increased and the antioxidant system was activated . Florasulam induced lipid peroxidation in larvae and adult zebrafish . It did not cause DNA damage to larvae but caused DNA damage to adult zebrafish .
Molecular Mechanism
Florasulam exerts its effects at the molecular level through several mechanisms. It binds to the ALS enzyme, inhibiting its activity and thereby preventing the synthesis of branched-chain amino acids . This inhibition disrupts protein synthesis and cell growth, leading to the death of the weed .
Temporal Effects in Laboratory Settings
The effects of Florasulam change over time in laboratory settings. The residues of Florasulam in wheat grain, wheat straw, and soil were below the detection limit . This suggests that Florasulam degrades over time in these environments .
Dosage Effects in Animal Models
The effects of Florasulam vary with different dosages in animal models. Florasulam has low or minimal acute toxicity via the oral, dermal, and inhalation routes of exposure . It is non-irritating to the eye and skin; it is not a skin sensitizer .
Metabolic Pathways
Florasulam is involved in several metabolic pathways. Metabolism of Florasulam was investigated in the cereal crop group following foliar application. Metabolic pathway suggests metabolisation of the parent compound via hydroxylation on the phenyl ring and subsequent glucose conjugation .
Transport and Distribution
Florasulam is transported and distributed within cells and tissues. Due to its high solubility in water, Florasulam can easily be transported within the plant system . It is also volatile, which allows it to be distributed in various parts of the plant .
Subcellular Localization
Given its role as an ALS inhibitor, it is likely that Florasulam localizes to the sites of ALS activity within the cell
特性
IUPAC Name |
N-(2,6-difluorophenyl)-8-fluoro-5-methoxy-[1,2,4]triazolo[1,5-c]pyrimidine-2-sulfonamide | |
---|---|---|
Source | PubChem | |
URL | https://pubchem.ncbi.nlm.nih.gov | |
Description | Data deposited in or computed by PubChem | |
InChI |
InChI=1S/C12H8F3N5O3S/c1-23-12-16-5-8(15)10-17-11(18-20(10)12)24(21,22)19-9-6(13)3-2-4-7(9)14/h2-5,19H,1H3 | |
Source | PubChem | |
URL | https://pubchem.ncbi.nlm.nih.gov | |
Description | Data deposited in or computed by PubChem | |
InChI Key |
QZXATCCPQKOEIH-UHFFFAOYSA-N | |
Source | PubChem | |
URL | https://pubchem.ncbi.nlm.nih.gov | |
Description | Data deposited in or computed by PubChem | |
Canonical SMILES |
COC1=NC=C(C2=NC(=NN21)S(=O)(=O)NC3=C(C=CC=C3F)F)F | |
Source | PubChem | |
URL | https://pubchem.ncbi.nlm.nih.gov | |
Description | Data deposited in or computed by PubChem | |
Molecular Formula |
C12H8F3N5O3S | |
Source | PubChem | |
URL | https://pubchem.ncbi.nlm.nih.gov | |
Description | Data deposited in or computed by PubChem | |
DSSTOX Substance ID |
DTXSID7044340 | |
Record name | Florasulam | |
Source | EPA DSSTox | |
URL | https://comptox.epa.gov/dashboard/DTXSID7044340 | |
Description | DSSTox provides a high quality public chemistry resource for supporting improved predictive toxicology. | |
Molecular Weight |
359.29 g/mol | |
Source | PubChem | |
URL | https://pubchem.ncbi.nlm.nih.gov | |
Description | Data deposited in or computed by PubChem | |
Solubility |
In n-heptane 0.019X10-3; xylene 0.227; n-octanol 0.184 (all in g/L solution, 20 °C). In dichloroethane 3.75; methanol 9.81; acetone 123; ethyl acetate 15.9; acetonitrile 72.1( all in g/L solvent, 20 °C), In water, 0.121 (purified, pH 5.6-5.8); 0.084 (pH 5.0); 6.36 (pH 7.0); 94.2 (pH 9) (all in g/L, 20 °C) | |
Record name | Florasulam | |
Source | Hazardous Substances Data Bank (HSDB) | |
URL | https://pubchem.ncbi.nlm.nih.gov/source/hsdb/7941 | |
Description | The Hazardous Substances Data Bank (HSDB) is a toxicology database that focuses on the toxicology of potentially hazardous chemicals. It provides information on human exposure, industrial hygiene, emergency handling procedures, environmental fate, regulatory requirements, nanomaterials, and related areas. The information in HSDB has been assessed by a Scientific Review Panel. | |
Density |
1.53 | |
Record name | Florasulam | |
Source | Hazardous Substances Data Bank (HSDB) | |
URL | https://pubchem.ncbi.nlm.nih.gov/source/hsdb/7941 | |
Description | The Hazardous Substances Data Bank (HSDB) is a toxicology database that focuses on the toxicology of potentially hazardous chemicals. It provides information on human exposure, industrial hygiene, emergency handling procedures, environmental fate, regulatory requirements, nanomaterials, and related areas. The information in HSDB has been assessed by a Scientific Review Panel. | |
Vapor Pressure |
1X10-2 mPa /7.501X10-8 mm Hg/ at 25 °C | |
Record name | Florasulam | |
Source | Hazardous Substances Data Bank (HSDB) | |
URL | https://pubchem.ncbi.nlm.nih.gov/source/hsdb/7941 | |
Description | The Hazardous Substances Data Bank (HSDB) is a toxicology database that focuses on the toxicology of potentially hazardous chemicals. It provides information on human exposure, industrial hygiene, emergency handling procedures, environmental fate, regulatory requirements, nanomaterials, and related areas. The information in HSDB has been assessed by a Scientific Review Panel. | |
Mechanism of Action |
Florasulam is a selective triazolopyrimidine sulfonanilide post-emergent herbicide. The pesticidal mode of action (MOA) is through inhibition of acetolactate synthase (ALS) in plants. ALS is found in the chloroplast where it catalyses branch chained amino acid biosynthesis. Inhibition of ALS results in inhibition of plant cell division, decreased plant growth, and ultimately, plant death. | |
Record name | Florasulam | |
Source | Hazardous Substances Data Bank (HSDB) | |
URL | https://pubchem.ncbi.nlm.nih.gov/source/hsdb/7941 | |
Description | The Hazardous Substances Data Bank (HSDB) is a toxicology database that focuses on the toxicology of potentially hazardous chemicals. It provides information on human exposure, industrial hygiene, emergency handling procedures, environmental fate, regulatory requirements, nanomaterials, and related areas. The information in HSDB has been assessed by a Scientific Review Panel. | |
Color/Form |
Solid | |
CAS RN |
145701-23-1 | |
Record name | Florasulam | |
Source | CAS Common Chemistry | |
URL | https://commonchemistry.cas.org/detail?cas_rn=145701-23-1 | |
Description | CAS Common Chemistry is an open community resource for accessing chemical information. Nearly 500,000 chemical substances from CAS REGISTRY cover areas of community interest, including common and frequently regulated chemicals, and those relevant to high school and undergraduate chemistry classes. This chemical information, curated by our expert scientists, is provided in alignment with our mission as a division of the American Chemical Society. | |
Explanation | The data from CAS Common Chemistry is provided under a CC-BY-NC 4.0 license, unless otherwise stated. | |
Record name | Florasulam [ISO] | |
Source | ChemIDplus | |
URL | https://pubchem.ncbi.nlm.nih.gov/substance/?source=chemidplus&sourceid=0145701231 | |
Description | ChemIDplus is a free, web search system that provides access to the structure and nomenclature authority files used for the identification of chemical substances cited in National Library of Medicine (NLM) databases, including the TOXNET system. | |
Record name | Florasulam | |
Source | DrugBank | |
URL | https://www.drugbank.ca/drugs/DB15316 | |
Description | The DrugBank database is a unique bioinformatics and cheminformatics resource that combines detailed drug (i.e. chemical, pharmacological and pharmaceutical) data with comprehensive drug target (i.e. sequence, structure, and pathway) information. | |
Explanation | Creative Common's Attribution-NonCommercial 4.0 International License (http://creativecommons.org/licenses/by-nc/4.0/legalcode) | |
Record name | Florasulam | |
Source | EPA DSSTox | |
URL | https://comptox.epa.gov/dashboard/DTXSID7044340 | |
Description | DSSTox provides a high quality public chemistry resource for supporting improved predictive toxicology. | |
Record name | [1,2,4]Triazolo[1,5-c]pyrimidine-2-sulfonamide, N-(2,6-difluorophenyl)-8-fluoro-5-methoxy | |
Source | European Chemicals Agency (ECHA) | |
URL | https://echa.europa.eu/substance-information/-/substanceinfo/100.127.229 | |
Description | The European Chemicals Agency (ECHA) is an agency of the European Union which is the driving force among regulatory authorities in implementing the EU's groundbreaking chemicals legislation for the benefit of human health and the environment as well as for innovation and competitiveness. | |
Explanation | Use of the information, documents and data from the ECHA website is subject to the terms and conditions of this Legal Notice, and subject to other binding limitations provided for under applicable law, the information, documents and data made available on the ECHA website may be reproduced, distributed and/or used, totally or in part, for non-commercial purposes provided that ECHA is acknowledged as the source: "Source: European Chemicals Agency, http://echa.europa.eu/". Such acknowledgement must be included in each copy of the material. ECHA permits and encourages organisations and individuals to create links to the ECHA website under the following cumulative conditions: Links can only be made to webpages that provide a link to the Legal Notice page. | |
Record name | FLORASULAM | |
Source | FDA Global Substance Registration System (GSRS) | |
URL | https://gsrs.ncats.nih.gov/ginas/app/beta/substances/00A64ZX8NB | |
Description | The FDA Global Substance Registration System (GSRS) enables the efficient and accurate exchange of information on what substances are in regulated products. Instead of relying on names, which vary across regulatory domains, countries, and regions, the GSRS knowledge base makes it possible for substances to be defined by standardized, scientific descriptions. | |
Explanation | Unless otherwise noted, the contents of the FDA website (www.fda.gov), both text and graphics, are not copyrighted. They are in the public domain and may be republished, reprinted and otherwise used freely by anyone without the need to obtain permission from FDA. Credit to the U.S. Food and Drug Administration as the source is appreciated but not required. | |
Record name | Florasulam | |
Source | Hazardous Substances Data Bank (HSDB) | |
URL | https://pubchem.ncbi.nlm.nih.gov/source/hsdb/7941 | |
Description | The Hazardous Substances Data Bank (HSDB) is a toxicology database that focuses on the toxicology of potentially hazardous chemicals. It provides information on human exposure, industrial hygiene, emergency handling procedures, environmental fate, regulatory requirements, nanomaterials, and related areas. The information in HSDB has been assessed by a Scientific Review Panel. | |
Melting Point |
220-221 °C (decomposes); also reported as 193.5-230.5 °C | |
Record name | Florasulam | |
Source | Hazardous Substances Data Bank (HSDB) | |
URL | https://pubchem.ncbi.nlm.nih.gov/source/hsdb/7941 | |
Description | The Hazardous Substances Data Bank (HSDB) is a toxicology database that focuses on the toxicology of potentially hazardous chemicals. It provides information on human exposure, industrial hygiene, emergency handling procedures, environmental fate, regulatory requirements, nanomaterials, and related areas. The information in HSDB has been assessed by a Scientific Review Panel. | |
Synthesis routes and methods
Procedure details
Retrosynthesis Analysis
AI-Powered Synthesis Planning: Our tool employs the Template_relevance Pistachio, Template_relevance Bkms_metabolic, Template_relevance Pistachio_ringbreaker, Template_relevance Reaxys, Template_relevance Reaxys_biocatalysis model, leveraging a vast database of chemical reactions to predict feasible synthetic routes.
One-Step Synthesis Focus: Specifically designed for one-step synthesis, it provides concise and direct routes for your target compounds, streamlining the synthesis process.
Accurate Predictions: Utilizing the extensive PISTACHIO, BKMS_METABOLIC, PISTACHIO_RINGBREAKER, REAXYS, REAXYS_BIOCATALYSIS database, our tool offers high-accuracy predictions, reflecting the latest in chemical research and data.
Strategy Settings
Precursor scoring | Relevance Heuristic |
---|---|
Min. plausibility | 0.01 |
Model | Template_relevance |
Template Set | Pistachio/Bkms_metabolic/Pistachio_ringbreaker/Reaxys/Reaxys_biocatalysis |
Top-N result to add to graph | 6 |
Feasible Synthetic Routes
Q & A
Q1: What is the primary mechanism of action of florasulam?
A1: Florasulam is a triazolopyrimidine sulfonanilide herbicide that acts by inhibiting the enzyme acetolactate synthase (ALS). [, ] This enzyme plays a crucial role in the biosynthesis of essential amino acids – valine, leucine, and isoleucine – in plants. []
Q2: How does the inhibition of ALS by florasulam affect susceptible plants?
A2: By inhibiting ALS, florasulam disrupts the production of these vital amino acids, leading to a halt in cell division and growth. This ultimately results in the death of the plant. [, , ]
Q3: What is the molecular formula and weight of florasulam?
A3: The molecular formula of florasulam is C18H18F5N5O3S, and its molecular weight is 463.44 g/mol. []
Q4: How does temperature affect the degradation of florasulam in soil?
A4: Research has shown that florasulam degradation in soil is influenced by temperature. Higher temperatures generally lead to faster degradation rates. [, ]
Q5: Does soil moisture content have a significant impact on florasulam degradation?
A5: Studies have found that soil moisture content has a negligible impact on the degradation rate of florasulam. []
Q6: How stable is florasulam during storage?
A6: Florasulam exhibits good storage stability. Residues in wheat grain and straw decreased by less than 30% after storage at -20°C for 365 days. []
Q7: How is florasulam absorbed and translocated in plants?
A7: Florasulam is primarily absorbed through the leaves and translocated through both the xylem and phloem to the growing points of the plant. []
Q8: How does the metabolism of florasulam differ between wheat and broadleaf weeds, contributing to its selectivity?
A8: Wheat metabolizes florasulam significantly faster than broadleaf weeds, contributing to its selectivity. The half-life of florasulam in wheat is around 2.4 hours, while in susceptible broadleaf weeds, it ranges from 19 to over 48 hours. []
Q9: What is the primary metabolic pathway of florasulam in wheat?
A9: In wheat, florasulam is primarily metabolized through hydroxylation of the aniline ring, followed by conjugation to glucose. []
Q10: What methods are used to evaluate the efficacy of florasulam in controlled environments?
A10: Greenhouse experiments are commonly used to evaluate the efficacy of florasulam. These experiments often involve dose-response studies to determine the herbicide's effect on plant growth and biomass. [, ]
Q11: Which weed species have been shown to be effectively controlled by florasulam in field trials?
A11: Field trials have demonstrated that florasulam effectively controls a wide range of broadleaf weeds, including cleavers (Galium aparine), chickweed (Stellaria media), corn poppy (Papaver rhoeas), and various cruciferous weeds. [, , ]
Q12: Has resistance to florasulam been observed in any weed species?
A12: Yes, resistance to florasulam, as well as cross-resistance to other ALS-inhibiting herbicides, has been reported in several weed species, including false cleavers (Galium spurium) and corn marigold (Glebionis segetum). [, , , ]
Q13: What are the known mechanisms of resistance to florasulam?
A13: The primary mechanism of resistance is target-site resistance, which involves mutations in the ALS gene. Common mutations leading to resistance include Pro-197-Thr and Trp-574-Leu substitutions. [, , , ]
Q14: What analytical techniques are commonly used for the detection and quantification of florasulam residues?
A14: Liquid chromatography coupled with tandem mass spectrometry (LC-MS/MS) is a widely used technique for the analysis of florasulam residues in various matrices, including plant tissues and soil. [, ]
Q15: What extraction and cleanup methods are employed for florasulam residue analysis?
A15: Common extraction methods include using solvents like acetonitrile. Cleanup procedures often involve solid-phase extraction (SPE) with cartridges containing materials like primary secondary amine (PSA), graphitized carbon black (GCB), and florisil to remove interfering compounds. [, ]
Q16: How quickly does florasulam degrade in soil?
A16: The half-life of florasulam in soil varies depending on factors like soil type, temperature, and pH. Studies have reported half-lives ranging from 2.4 to 24.8 days. [, , , ]
Q17: What are the major degradation pathways of florasulam in the environment?
A17: Photodegradation, primarily through exposure to sunlight, plays a significant role in the degradation of florasulam in the environment. [] Microbial degradation is also a contributing factor. []
Q18: What other herbicides or weed management strategies can be used as alternatives to florasulam, especially considering the emergence of resistance?
A24: Integrated weed management strategies that incorporate diverse herbicide modes of action are crucial to manage resistance. Alternatives to florasulam include herbicides with different modes of action, such as synthetic auxins like 2,4-D, bromoxynil, or clopyralid, as well as herbicides from other chemical classes like pyroxsulam, fluroxypyr, and halauxifen-methyl. [, , , , , , , , , , ] Additionally, non-chemical weed control methods, such as mechanical weeding and crop rotation, can be integrated to further reduce reliance on specific herbicides. []
試験管内研究製品の免責事項と情報
BenchChemで提示されるすべての記事および製品情報は、情報提供を目的としています。BenchChemで購入可能な製品は、生体外研究のために特別に設計されています。生体外研究は、ラテン語の "in glass" に由来し、生物体の外で行われる実験を指します。これらの製品は医薬品または薬として分類されておらず、FDAから任何の医療状態、病気、または疾患の予防、治療、または治癒のために承認されていません。これらの製品を人間または動物に体内に導入する形態は、法律により厳格に禁止されています。これらのガイドラインに従うことは、研究と実験において法的および倫理的な基準の遵守を確実にするために重要です。