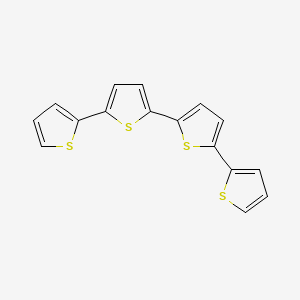
2,2':5',2'':5'',2'''-Quaterthiophene
概要
説明
2,2’:5’,2’‘:5’‘,2’‘’-Quaterthiophene is a derivative of quarterthiophene that can be used as a donor-acceptor molecule in organic electronic devices . It has a field mobility of 0.23 cm^2/Vs and acts as an active layer in semiconductors . Another derivative, 5,5′′′-Bis (tridecafluorohexyl)-2,2′:5′,2′′:5′′,2′′′-quaterthiophene (DFH-4T), is a n-type semiconducting material that can be used as an electron transporting layer .
Synthesis Analysis
The synthesis of 2,2’:5’,2’‘:5’‘,2’‘’-Quaterthiophene involves the reaction of bromosuccinimide with esters of 3-substituted 2,2’-bithiophene-5-carboxylic acids to obtain their 5’-bromo derivatives . These are further converted to esters of 3,3’‘’-disubstituted 2,2’:5’,2’‘:5’‘,2’‘’-quaterthiophene-5,5’‘’-dicarboxylic acids by heating in DMF with catalytic amounts of Pd (PPh 3) 4 .Molecular Structure Analysis
The molecular structure of 2,2’:5’,2’‘:5’‘,2’‘’-Quaterthiophene is represented by the empirical formula C16H10S4 . Its molecular weight is 330.51 .Chemical Reactions Analysis
The chemical reactions involved in the synthesis of 2,2’:5’,2’‘:5’‘,2’‘’-Quaterthiophene include the reaction of bromosuccinimide with esters of 3-substituted 2,2’-bithiophene-5-carboxylic acids . This is followed by a reaction with ethyl thioglycolate in the presence of sodium ethoxide .Physical And Chemical Properties Analysis
2,2’:5’,2’‘:5’‘,2’‘’-Quaterthiophene has a melting point of 211-214 °C (lit.) . It exhibits p-type semiconductor properties with a mobility of 1.2×10−3 cm^2/V·s .科学的研究の応用
Excited States and Spectroscopic Features
- Excited States Analysis : A study by Rubio, Merchán, and Ortí (2005) explored the low-lying singlet and triplet valence excited states of 2,2':5',2'':5'',2'''-quaterthiophene. Their high-level ab initio computations provided insights into the spectroscopic features of short alpha-oligothiophenes, emphasizing the nonradiative decay process through intersystem crossing (ISC) from singlet to triplet states, notably less efficient in quaterthiophene (Rubio, Merchán, & Ortí, 2005).
Electronic and Optical Properties
- Thin-Film Transistors : Yoon et al. (2005) described the synthesis and characterization of new carbonyl-functionalized quaterthiophenes, highlighting their application in field-effect transistors with high electron mobilities and excellent ambipolar transport. This research underscores quaterthiophene's potential in organic complementary circuits (Yoon, Dibenedetto, Facchetti, & Marks, 2005).
- Vibrational and Geometric Structures : The correlation between vibrational spectra and electrical conductivity of polythiophene, including quaterthiophene, was investigated by Akimoto et al. (1986). They analyzed the vibrational spectra of polythiophene films and model compounds, revealing that a high degree of polymerization and the abundance of long conjugated coplanar segments are crucial for high electrical conductivity (Akimoto, Furukawa, Takeuchi, Harada, Soma, & Sōma, 1986).
Photophysical and Structural Analysis
- Optical Properties and Conformational Changes : Breza, Lukes, and Vrábel (2001) conducted a study on the dependence of optical properties on conformational changes in oligothiophenes, including quaterthiophene. They optimized geometries using the AM1 method and calculated electron spectra, contributing to the understanding of how torsional angles impact the electronic structure of oligothiophenes (Breza, Lukes, & Vrábel, 2001).
- Ultrafast Structural Relaxation in Solution : Zhou, Yu, and Bragg (2015) used femtosecond stimulated Raman spectroscopy to study the ultrafast structural relaxation of photoexcited quaterthiophene in solution. Their research highlights the importance of intraring C═C stretching features in understanding the dynamics of photoexcited conjugated materials (Zhou, Yu, & Bragg, 2015).
将来の方向性
特性
IUPAC Name |
2-thiophen-2-yl-5-(5-thiophen-2-ylthiophen-2-yl)thiophene | |
---|---|---|
Source | PubChem | |
URL | https://pubchem.ncbi.nlm.nih.gov | |
Description | Data deposited in or computed by PubChem | |
InChI |
InChI=1S/C16H10S4/c1-3-11(17-9-1)13-5-7-15(19-13)16-8-6-14(20-16)12-4-2-10-18-12/h1-10H | |
Source | PubChem | |
URL | https://pubchem.ncbi.nlm.nih.gov | |
Description | Data deposited in or computed by PubChem | |
InChI Key |
FXEJOIFDICYSSO-UHFFFAOYSA-N | |
Source | PubChem | |
URL | https://pubchem.ncbi.nlm.nih.gov | |
Description | Data deposited in or computed by PubChem | |
Canonical SMILES |
C1=CSC(=C1)C2=CC=C(S2)C3=CC=C(S3)C4=CC=CS4 | |
Source | PubChem | |
URL | https://pubchem.ncbi.nlm.nih.gov | |
Description | Data deposited in or computed by PubChem | |
Molecular Formula |
C16H10S4 | |
Source | PubChem | |
URL | https://pubchem.ncbi.nlm.nih.gov | |
Description | Data deposited in or computed by PubChem | |
Related CAS |
103945-18-2 | |
Record name | 2,2′:5′,2′′:5′′,2′′′-Quaterthiophene, homopolymer | |
Source | CAS Common Chemistry | |
URL | https://commonchemistry.cas.org/detail?cas_rn=103945-18-2 | |
Description | CAS Common Chemistry is an open community resource for accessing chemical information. Nearly 500,000 chemical substances from CAS REGISTRY cover areas of community interest, including common and frequently regulated chemicals, and those relevant to high school and undergraduate chemistry classes. This chemical information, curated by our expert scientists, is provided in alignment with our mission as a division of the American Chemical Society. | |
Explanation | The data from CAS Common Chemistry is provided under a CC-BY-NC 4.0 license, unless otherwise stated. | |
DSSTOX Substance ID |
DTXSID40204863 | |
Record name | 2,2':5',2'',5'',2'''-Quaterthiophene | |
Source | EPA DSSTox | |
URL | https://comptox.epa.gov/dashboard/DTXSID40204863 | |
Description | DSSTox provides a high quality public chemistry resource for supporting improved predictive toxicology. | |
Molecular Weight |
330.5 g/mol | |
Source | PubChem | |
URL | https://pubchem.ncbi.nlm.nih.gov | |
Description | Data deposited in or computed by PubChem | |
Product Name |
2,2':5',2'':5'',2'''-Quaterthiophene | |
CAS RN |
5632-29-1 | |
Record name | 2,2':5',2'',5'',2'''-Quaterthiophene | |
Source | ChemIDplus | |
URL | https://pubchem.ncbi.nlm.nih.gov/substance/?source=chemidplus&sourceid=0005632291 | |
Description | ChemIDplus is a free, web search system that provides access to the structure and nomenclature authority files used for the identification of chemical substances cited in National Library of Medicine (NLM) databases, including the TOXNET system. | |
Record name | 2,2':5',2'',5'',2'''-Quaterthiophene | |
Source | EPA DSSTox | |
URL | https://comptox.epa.gov/dashboard/DTXSID40204863 | |
Description | DSSTox provides a high quality public chemistry resource for supporting improved predictive toxicology. | |
Synthesis routes and methods I
Procedure details
Synthesis routes and methods II
Procedure details
Retrosynthesis Analysis
AI-Powered Synthesis Planning: Our tool employs the Template_relevance Pistachio, Template_relevance Bkms_metabolic, Template_relevance Pistachio_ringbreaker, Template_relevance Reaxys, Template_relevance Reaxys_biocatalysis model, leveraging a vast database of chemical reactions to predict feasible synthetic routes.
One-Step Synthesis Focus: Specifically designed for one-step synthesis, it provides concise and direct routes for your target compounds, streamlining the synthesis process.
Accurate Predictions: Utilizing the extensive PISTACHIO, BKMS_METABOLIC, PISTACHIO_RINGBREAKER, REAXYS, REAXYS_BIOCATALYSIS database, our tool offers high-accuracy predictions, reflecting the latest in chemical research and data.
Strategy Settings
Precursor scoring | Relevance Heuristic |
---|---|
Min. plausibility | 0.01 |
Model | Template_relevance |
Template Set | Pistachio/Bkms_metabolic/Pistachio_ringbreaker/Reaxys/Reaxys_biocatalysis |
Top-N result to add to graph | 6 |
Feasible Synthetic Routes
Q & A
Q1: What is the molecular formula and weight of quaterthiophene?
A1: The molecular formula of quaterthiophene is C16H10S4, and its molecular weight is 322.51 g/mol. []
Q2: How does the introduction of carbonyl groups affect the structure of quaterthiophene?
A2: Theoretical calculations show that introducing carbonyl groups to the quaterthiophene backbone induces progressive quinoidization compared to the non-functionalized molecule. This effect is further amplified when combined with perfluorohexyl chains. []
Q3: What is the significance of the strong Raman line observed in the Raman spectra of carbonyl-functionalized quaterthiophenes?
A3: The prominent Raman line primarily represents a combination of C-C/C=C stretching vibrations across the entire thiophene core. This band exhibits a significant downshift due to structural changes caused by the electron-withdrawing carbonyl groups on the π-conjugated backbone. []
Q4: What structural information can be obtained from the Raman spectra of carbonyl-bridged quaterthiophenes?
A4: The splitting of the dominant Raman band upon incorporating a central carbonyl bridge indicates the formation of two distinct structural domains within the molecule. []
Q5: How does functionalization with electron-withdrawing groups affect the optical properties of quaterthiophene?
A5: Functionalization with carbonyl groups and perfluorohexyl chains leads to a noticeable bathochromic shift in both optical absorption and fluorescence bands. This shift suggests an expansion of the π-conjugation within the molecule. []
Q6: How does the introduction of long alkyl side chains impact the solubility and self-assembly of quaterthiophene derivatives?
A6: Long alkyl side chains enhance the solubility of quaterthiophene derivatives in organic solvents and promote self-assembly. []
Q7: What type of aggregates do alkyl-substituted quaterthiophenes typically form in solution?
A7: UV/Vis absorption measurements indicate that alkyl-substituted quaterthiophenes tend to form H-type aggregates, characterized by face-to-face packing structures. []
Q8: What electronic properties make quaterthiophene and its derivatives suitable for organic field-effect transistors (OFETs)?
A8: Quaterthiophene derivatives demonstrate favorable properties for OFETs, including high carrier mobility, large current on/off ratios, and air stability in specific configurations. [, , , , ]
Q9: How does the molecular structure of quaterthiophene derivatives influence their performance in OFETs?
A9: The molecular structure significantly influences OFET performance. For instance, asymmetric thiophene-naphthalene oligomers exhibit varying mobilities depending on the degree of π overlap and morphological differences dictated by their structure. []
Q10: How does the presence of fluorine atoms in the structure affect the electronic properties of quaterthiophene derivatives?
A10: Fluorocarbon-modified quaterthiophenes, particularly those with perfluorophenyl substitutions, often exhibit n-type semiconducting behavior, making them suitable for n-channel OFETs. []
Q11: What strategies can be employed to achieve air-stable n-channel OFET operation using quaterthiophene derivatives?
A11: Incorporating electron-deficient units like quinones or perfluorophenacyl groups into the quaterthiophene structure can result in air-stable n-channel OFET performance. [, ]
Q12: What unique morphological feature has been observed in thin films of certain quaterthiophene-based polymers?
A12: Some quaterthiophene-based polymers, like poly(N-(2-octyldodecyl)-2,2'-bithiophene-3,3'-dicarboximide), exhibit terracing in AFM images after annealing. The step height in these terraces corresponds to the d spacing observed in X-ray diffraction, a rare phenomenon in polymeric organic semiconductors. []
Q13: How does the alkyl side chain length in poly(3-alkylthiophene)s affect their performance in electrolyte-gated transistors (EGTs)?
A13: Increasing the alkyl side chain length in poly(3-alkylthiophene)s leads to enhanced carrier mobilities and reduced hysteresis in their conductivity peaks when incorporated into EGTs. []
Q14: What is the significance of the conductivity peak observed at a specific hole density in polythiophene-based EGTs?
A14: The conductivity peak, consistently observed at a hole density of approximately 0.4 holes per thiophene ring across various polythiophenes, suggests the complete filling of a polaronic sub-band. Beyond this point, the hole mobility collapses, leading to conductivity suppression. []
Q15: What theoretical methods are commonly used to study the electronic and optical properties of quaterthiophene derivatives?
A15: Density functional theory (DFT), time-dependent DFT (TDDFT), and ab initio Hartree-Fock calculations are frequently employed to investigate the ground and excited state properties of these molecules. [, , ]
Q16: How does the chain length of oligothiophenes affect their electronic properties, as predicted by theoretical calculations?
A16: Theoretical studies indicate that increasing the thiophene chain length generally leads to a decrease in the energy gap (HOMO-LUMO gap) of oligothiophenes. [, ]
Q17: What information can be obtained from theoretical calculations regarding the charge transport properties of quaterthiophene derivatives?
A17: Calculations can predict parameters like reorganization energy, ionization potential, and electron affinity, offering insights into the charge transport behavior of these molecules. [, ]
試験管内研究製品の免責事項と情報
BenchChemで提示されるすべての記事および製品情報は、情報提供を目的としています。BenchChemで購入可能な製品は、生体外研究のために特別に設計されています。生体外研究は、ラテン語の "in glass" に由来し、生物体の外で行われる実験を指します。これらの製品は医薬品または薬として分類されておらず、FDAから任何の医療状態、病気、または疾患の予防、治療、または治癒のために承認されていません。これらの製品を人間または動物に体内に導入する形態は、法律により厳格に禁止されています。これらのガイドラインに従うことは、研究と実験において法的および倫理的な基準の遵守を確実にするために重要です。