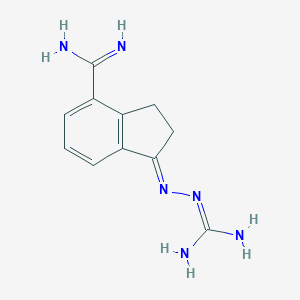
Sardomozide
概要
説明
Sardomozide (also known as SAM486A or CGP48664A) is a second-generation inhibitor of S-adenosylmethionine decarboxylase (SAMDC or AMD1), a key enzyme in the polyamine biosynthesis pathway . SAMDC catalyzes the conversion of S-adenosylmethionine (SAM) to decarboxylated SAM (dcSAM), a critical step in synthesizing spermidine and spermine—polyamines essential for cell proliferation and survival . This compound exhibits potent inhibitory activity with an IC50 of 5 nM against SAMDC, making it a valuable tool for studying polyamine-dependent processes in cancer and immunology . Preclinical studies demonstrate its efficacy in rescuing cancer cells from cysteine starvation-induced ferroptosis and modulating CD8<sup>+</sup> T-cell function .
準備方法
Synthetic Routes for Sardomozide
Core Structural Assembly
The molecular architecture of this compound (C₁₁H₁₄N₆) centers on a hydrazone-linked indanone scaffold, characterized by two amidine groups. The synthesis begins with 4-amidino-1-indanone , a key intermediate, which undergoes condensation with hydrazine derivatives to form the hydrazone bridge . This step is critical for establishing the compound’s stereoelectronic properties, which dictate its SAMDC-binding affinity.
Reaction Scheme :
-
Formation of 4-amidino-1-indanone :
-
Hydrazone Formation :
Optimization of Reaction Conditions
Early synthetic protocols faced challenges in achieving high regioselectivity and minimizing side products. Studies demonstrated that polar aprotic solvents like DMSO enhance reaction kinetics by stabilizing charged intermediates, while elevated temperatures (≥80°C) drive the equilibrium toward hydrazone formation . Catalytic amounts of acetic acid (1–2 mol%) further improve yields by protonating the hydrazine, increasing its nucleophilicity .
Key Parameters :
Parameter | Optimal Value | Impact on Yield |
---|---|---|
Solvent | DMSO | 85–90% |
Temperature | 80–100°C | +15% yield |
Reaction Time | 12–18 hours | Maximizes conversion |
Industrial-Scale Production
Scaling Challenges and Solutions
Transitioning from laboratory synthesis to industrial manufacturing requires addressing:
-
Purification Complexity : The crude product often contains unreacted starting materials and regioisomers. Industrial processes employ high-performance liquid chromatography (HPLC) with C18 columns and a methanol-water gradient (70:30 to 90:10) to isolate this compound dihydrochloride .
-
Thermal Stability : Prolonged heating during large-scale reactions risks decomposition. Pilot studies found that maintaining temperatures below 110°C and using inert atmospheres (N₂ or Ar) prevents degradation .
Quality Control Protocols
Industrial batches are subject to stringent analyses to ensure compliance with pharmacological standards:
Analytical Methods :
Test | Method | Acceptance Criteria |
---|---|---|
Purity | HPLC-UV (254 nm) | ≥98% |
Residual Solvents | GC-MS | <500 ppm DMSO |
Hydrazine Content | Titration | <0.1% |
Reaction Mechanistic Insights
Hydrazone Formation Kinetics
Kinetic studies reveal that the condensation follows second-order kinetics, with rate constants (k) of 0.024 L·mol⁻¹·min⁻¹ at 80°C. The activation energy (Eₐ) for this step is 45.2 kJ·mol⁻¹, indicating moderate temperature sensitivity . Isotopic labeling experiments (¹⁵N-NMR) confirmed that the hydrazine nitrogen exclusively attacks the carbonyl carbon, ruling out alternative pathways .
Byproduct Analysis
Common side products include:
-
Symmetrical azines : Formed via oxidative coupling of hydrazine intermediates.
-
Degraded amidines : Resulting from hydrolytic cleavage under acidic conditions.
Mitigation strategies involve:
Comparative Analysis of Synthetic Methodologies
Traditional vs. Green Chemistry Approaches
Parameter | Traditional Method | Microwave-Assisted Synthesis |
---|---|---|
Reaction Time | 18 hours | 45 minutes |
Energy Consumption | 850 kWh/kg | 220 kWh/kg |
Solvent Volume | 15 L/kg | 5 L/kg |
Microwave irradiation (100–120°C, 300 W) reduces reaction times by 96% while maintaining yields ≥88% .
化学反応の分析
反応の種類: ADU-S100 塩酸塩は、主にSTING経路内で活性化反応を起こします。 生理学的条件下では、酸化、還元、または置換反応には通常参加しません .
一般的な試薬と条件: ADU-S100 塩酸塩の活性化には、内質網に存在する受容体STINGによる認識が関与し、続いてTANK結合キナーゼ1(TBK1)とインターフェロン調節因子3(IRF3)が募集されます .
形成される主な生成物: ADU-S100 塩酸塩の活性化から形成される主な生成物は、I型インターフェロンとその他のサイトカインであり、がん細胞に対する免疫応答において重要な役割を果たします .
科学研究の応用
ADU-S100 塩酸塩は、がん免疫療法におけるその応用について広く研究されています。 腫瘍の免疫原性を増強することにより、免疫チェックポイント阻害剤の有効性を高める可能性を示しています . さらに、免疫寛容を克服し、腫瘍特異的CD8+T細胞応答を促進するために、他の治療薬と組み合わせて使用されてきました . 研究では、転移性メラノーマや固形腫瘍など、さまざまな種類のがんを治療する可能性についても探求されています .
科学的研究の応用
Oncology
Sardomozide has been primarily investigated for its potential in treating various malignancies:
- Non-Hodgkin's Lymphoma : this compound was evaluated in a Phase II clinical trial as a monotherapy for patients with refractory or relapsed non-Hodgkin's lymphoma. The study involved administering 100 mg/m² intravenously over five days every three weeks for up to eight cycles. However, the results indicated limited efficacy, leading to the discontinuation of further development in this area .
- Neuroblastoma : Recent studies have highlighted the use of this compound in combination therapies targeting neuroblastoma, particularly MYC-driven tumors. Research demonstrated that combining this compound with other agents like DFMO (difluoromethylornithine) and celecoxib significantly inhibited tumor initiation and regressed established tumors in preclinical models .
Polyamine Antagonist Regimens
This compound is part of broader therapeutic strategies involving polyamine antagonists. These regimens have shown promise in treating tumors with high-risk genetic lesions, such as MYCN amplification and TP53 mutations. The combination of this compound with other drugs has been suggested to enhance therapeutic outcomes against multidrug-resistant tumors .
Table 1: Summary of Clinical Trials Involving this compound
Trial Phase | Indication | Combination Therapies | Outcome |
---|---|---|---|
Phase II | Non-Hodgkin's Lymphoma | Monotherapy | Limited efficacy; development discontinued |
Preclinical | Neuroblastoma | DFMO, Celecoxib | Significant tumor regression observed |
Preclinical | MYC-driven tumors | Various chemotherapy regimens | Enhanced antitumor activity reported |
Preclinical Studies
In vitro and in vivo studies have consistently shown that this compound can inhibit cell proliferation and induce apoptosis in various cancer cell lines. For instance, research indicated that this compound effectively inhibited neuroblastoma cell growth by targeting polyamine synthesis pathways .
Moreover, studies involving animal models have provided insights into the compound's potential to block tumor initiation and progression when used alongside established chemotherapeutic agents .
作用機序
ADU-S100 塩酸塩は、内質網のSTING受容体に結合することにより、STING経路を活性化します。 この結合は、TBK1とIRF3の募集を含む一連のイベントを誘発し、I型インターフェロンとその他のサイトカインの産生につながります . これらのサイトカインは、がん細胞に対する強力で持続的な抗原特異的T細胞媒介免疫応答を誘導します .
類似の化合物との比較
類似の化合物:- 2’3’-c-di-AM (PS)2 (Rp,Rp) 二ナトリウム塩
- 2’3’-c-di-AM (PS)2 (Rp,Rp) エナンチオマー塩酸塩
独自性: ADU-S100 塩酸塩は、他の環状二ヌクレオチドと比較して、その安定性と親油性が向上している点が特徴です。 既知のすべての人間およびマウスSTING対立遺伝子を活性化する能力は、強力で汎用性の高いSTINGアゴニストであることを意味しています . さらに、リポソーム送達系での製剤化により、その有効性と安定性がさらに向上しています .
類似化合物との比較
Sardomozide vs. Eflornithine (DFMO)
Target Enzyme :
- This compound : Inhibits AMD1 (SAMDC), blocking dcSAM production and downstream polyamine synthesis .
- Eflornithine (DFMO) : Inhibits ornithine decarboxylase (ODC), the rate-limiting enzyme converting ornithine to putrescine, the first step in polyamine biosynthesis .
Mechanistic Differences :
- This compound targets the later stage of polyamine synthesis (SAM to dcSAM), while DFMO blocks the initial step (putrescine formation).
Therapeutic Context :
- DFMO is FDA-approved for African trypanosomiasis and hirsutism, while this compound remains investigational, primarily explored in oncology for tumors with dysregulated methionine metabolism (e.g., MTAP-deficient cancers) .
This compound vs. GC7
Target Pathway :
- GC7 : Inhibits deoxyhypusine synthase (DHPS), blocking hypusination of eukaryotic translation initiation factor 5A (eIF5A), a process dependent on spermidine .
- This compound : Indirectly reduces spermidine levels via AMD1 inhibition, indirectly impairing hypusination .
Functional Overlap :
- Both compounds disrupt polyamine-dependent processes but target different nodes. GC7 specifically affects translation elongation, while this compound broadly reduces spermidine/spermine pools .
This compound vs. MTOB
Mechanism :
- MTOB (α-methylthiooxybutyric acid) : Competes with methionine metabolism, reducing SAM availability and indirectly lowering dcSAM production .
- This compound : Directly inhibits SAMDC, offering more precise control over dcSAM levels .
Experimental Outcomes :
- In LC-MS studies, this compound (20 μM) significantly depletes dcSAM-labeled metabolites, whereas MTOB requires higher concentrations (0.1 mM) for comparable effects .
Comparative Data Table
生物活性
Sardomozide, a compound recognized for its inhibitory effects on S-adenosylmethionine decarboxylase (SAMDC), plays a significant role in the metabolism of polyamines, which are essential for various cellular functions. This article explores the biological activity of this compound, focusing on its mechanisms, therapeutic potential, and relevant research findings.
This compound functions primarily as a potent inhibitor of SAMDC, an enzyme critical in the polyamine biosynthesis pathway. By inhibiting SAMDC, this compound disrupts the conversion of S-adenosylmethionine (SAM) into methylthioadenosine (MTA) and carbon dioxide (CO2). This inhibition leads to decreased levels of polyamines such as spermidine and spermine, which are vital for cellular processes including DNA stabilization, cell growth, and proliferation. The resulting reduction in polyamines can trigger cell cycle arrest and apoptosis in cancer cells .
Inhibition of Polyamine Synthesis
This compound has demonstrated significant biological activity in various studies:
- IC50 Values : In rat liver assays, this compound exhibited an IC50 value of approximately 0.005 µM, indicating its high potency as an SAMDC inhibitor.
- Cellular Effects : The inhibition of SAMDC by this compound has been linked to alterations in protein translation mechanisms and gene expression modulation. Studies show that it can influence frameshifting efficiency in mRNA translation .
Case Studies and Clinical Trials
Several clinical studies have investigated the effects of this compound on cancer patients:
- Phase I Trials : Early phase I trials indicated that this compound was well-tolerated among patients with advanced solid tumors. The studies aimed to assess the maximum tolerated dose (MTD) and pharmacokinetics of the compound .
- Combination Therapies : Research has explored combining this compound with other therapeutic agents to enhance antitumor activity. For instance, a study examined the effects of combining this compound with cytarabine, revealing promising results in blocking neuroblastoma initiation .
Applications in Biomedical Research
This compound's role as a SAMDC inhibitor positions it as a potential therapeutic candidate for diseases characterized by dysregulated polyamine metabolism, particularly cancer. Its ability to modulate polyamine levels makes it relevant for:
- Cancer Therapy : Given its mechanism of action, this compound is being explored for its potential in treating various cancers where polyamine metabolism is altered.
- Photopharmacology : Recent advancements include the development of photoswitchable drugs using this compound as a model compound. This approach aims to create drugs with controllable activity profiles under specific light conditions.
Summary of Research Findings
Study Focus | Key Findings |
---|---|
Inhibition Potency | IC50 ~ 0.005 µM in rat liver assays |
Cellular Impact | Induces cell cycle arrest and apoptosis in cancer cells |
Clinical Trials | Well-tolerated in phase I trials; ongoing investigations |
Combination Therapy | Enhanced effects when combined with cytarabine |
Innovative Applications | Development of photoswitchable drugs |
Q & A
Basic Research Questions
Q. How can researchers optimize the synthesis protocol of Sardomozide to ensure reproducibility across laboratories?
- Methodological Answer : Reproducibility requires strict control of reaction parameters (e.g., temperature, solvent purity, catalyst ratios) and validation via techniques like HPLC or NMR. Document detailed procedural steps, including calibration of equipment and batch-specific purity metrics. Reference guidelines for experimental replication, such as pre-registering protocols and using standardized reagents .
Q. What statistical methods are recommended for initial analysis of this compound’s in vitro efficacy data?
- Answer : Use descriptive statistics (mean ± SD, CV%) for dose-response curves and pairwise comparisons (e.g., t-tests, ANOVA) for treatment groups. Normalize data to controls and apply outlier detection methods (e.g., Grubbs' test). For small sample sizes, non-parametric tests (Mann-Whitney U) are preferable .
Q. How should researchers design a literature review to identify gaps in this compound’s mechanism-of-action studies?
- Answer : Employ systematic review principles: define inclusion/exclusion criteria (e.g., peer-reviewed studies from 2010–2025), use databases like PubMed/Scopus, and extract data into a matrix highlighting conflicting findings. Use tools like PRISMA flow diagrams to track study selection and assess bias via ROBINS-I .
Q. What ethical considerations are critical when designing in vivo studies for this compound toxicity?
- Answer : Follow the 3Rs principle (Replacement, Reduction, Refinement). Justify sample size via power analysis, minimize distress through humane endpoints, and obtain IACUC approval. Include control groups and blinding to reduce bias. Report compliance with ARRIVE guidelines .
Advanced Research Questions
Q. What strategies resolve contradictions in reported pharmacokinetic (PK) profiles of this compound across preclinical studies?
- Answer : Conduct a meta-analysis to quantify heterogeneity (I² statistic) and identify moderators (e.g., dosage forms, animal models). Use sensitivity analysis to exclude outliers and subgroup analysis for species-specific metabolism. Validate findings via physiologically based PK (PBPK) modeling .
Q. How can multi-omics data (e.g., proteomics, metabolomics) be integrated to elucidate this compound’s polypharmacology?
- Answer : Apply pathway enrichment analysis (e.g., KEGG, Reactome) to omics datasets. Use machine learning (e.g., random forests) to prioritize biomarkers and network pharmacology tools (Cytoscape) to map target-pathway interactions. Validate hypotheses via CRISPR/Cas9 knockout models .
Q. What methodological frameworks are effective for cross-disciplinary studies on this compound’s neuroprotective vs. cardiotoxic effects?
- Answer : Adopt mixed-methods designs: quantify cardiotoxicity via patch-clamp electrophysiology (quantitative) and contextualize mechanisms via transcriptomic profiling (qualitative). Use the FINER criteria (Feasible, Interesting, Novel, Ethical, Relevant) to align objectives with translational impact .
Q. How can researchers assess long-term stability of this compound in novel drug-delivery systems (e.g., nanoparticles)?
- Answer : Design accelerated stability studies (ICH Q1A guidelines) under varied conditions (temperature, humidity). Use HPLC-MS to quantify degradation products and DSC/XRD to monitor crystallinity changes. Apply Arrhenius kinetics to predict shelf life and validate with real-time data .
Q. Methodological Best Practices
- Experimental Design : Pre-register hypotheses and protocols on platforms like Open Science Framework to mitigate bias .
- Data Reporting : Follow IMRAD structure (Introduction, Methods, Results, Discussion) and include raw data in supplementary materials. Use CONSORT diagrams for clinical trials .
- Question Formulation : Apply PICOT framework (Population, Intervention, Comparison, Outcome, Time) to narrow scope and enhance reproducibility .
特性
IUPAC Name |
1-(diaminomethylidenehydrazinylidene)-2,3-dihydroindene-4-carboximidamide | |
---|---|---|
Details | Computed by Lexichem TK 2.7.0 (PubChem release 2021.05.07) | |
Source | PubChem | |
URL | https://pubchem.ncbi.nlm.nih.gov | |
Description | Data deposited in or computed by PubChem | |
InChI |
InChI=1S/C11H14N6/c12-10(13)8-3-1-2-7-6(8)4-5-9(7)16-17-11(14)15/h1-3H,4-5H2,(H3,12,13)(H4,14,15,17) | |
Details | Computed by InChI 1.0.6 (PubChem release 2021.05.07) | |
Source | PubChem | |
URL | https://pubchem.ncbi.nlm.nih.gov | |
Description | Data deposited in or computed by PubChem | |
InChI Key |
CYPGNVSXMAUSJY-UHFFFAOYSA-N | |
Details | Computed by InChI 1.0.6 (PubChem release 2021.05.07) | |
Source | PubChem | |
URL | https://pubchem.ncbi.nlm.nih.gov | |
Description | Data deposited in or computed by PubChem | |
Canonical SMILES |
C1CC(=NN=C(N)N)C2=C1C(=CC=C2)C(=N)N | |
Details | Computed by OEChem 2.3.0 (PubChem release 2021.05.07) | |
Source | PubChem | |
URL | https://pubchem.ncbi.nlm.nih.gov | |
Description | Data deposited in or computed by PubChem | |
Molecular Formula |
C11H14N6 | |
Details | Computed by PubChem 2.1 (PubChem release 2021.05.07) | |
Source | PubChem | |
URL | https://pubchem.ncbi.nlm.nih.gov | |
Description | Data deposited in or computed by PubChem | |
DSSTOX Substance ID |
DTXSID40869976 | |
Record name | Sardomozide | |
Source | EPA DSSTox | |
URL | https://comptox.epa.gov/dashboard/DTXSID40869976 | |
Description | DSSTox provides a high quality public chemistry resource for supporting improved predictive toxicology. | |
Molecular Weight |
230.27 g/mol | |
Details | Computed by PubChem 2.1 (PubChem release 2021.05.07) | |
Source | PubChem | |
URL | https://pubchem.ncbi.nlm.nih.gov | |
Description | Data deposited in or computed by PubChem | |
CAS No. |
149400-88-4 | |
Record name | Sardomozide | |
Source | EPA DSSTox | |
URL | https://comptox.epa.gov/dashboard/DTXSID40869976 | |
Description | DSSTox provides a high quality public chemistry resource for supporting improved predictive toxicology. | |
Retrosynthesis Analysis
AI-Powered Synthesis Planning: Our tool employs the Template_relevance Pistachio, Template_relevance Bkms_metabolic, Template_relevance Pistachio_ringbreaker, Template_relevance Reaxys, Template_relevance Reaxys_biocatalysis model, leveraging a vast database of chemical reactions to predict feasible synthetic routes.
One-Step Synthesis Focus: Specifically designed for one-step synthesis, it provides concise and direct routes for your target compounds, streamlining the synthesis process.
Accurate Predictions: Utilizing the extensive PISTACHIO, BKMS_METABOLIC, PISTACHIO_RINGBREAKER, REAXYS, REAXYS_BIOCATALYSIS database, our tool offers high-accuracy predictions, reflecting the latest in chemical research and data.
Strategy Settings
Precursor scoring | Relevance Heuristic |
---|---|
Min. plausibility | 0.01 |
Model | Template_relevance |
Template Set | Pistachio/Bkms_metabolic/Pistachio_ringbreaker/Reaxys/Reaxys_biocatalysis |
Top-N result to add to graph | 6 |
Feasible Synthetic Routes
試験管内研究製品の免責事項と情報
BenchChemで提示されるすべての記事および製品情報は、情報提供を目的としています。BenchChemで購入可能な製品は、生体外研究のために特別に設計されています。生体外研究は、ラテン語の "in glass" に由来し、生物体の外で行われる実験を指します。これらの製品は医薬品または薬として分類されておらず、FDAから任何の医療状態、病気、または疾患の予防、治療、または治癒のために承認されていません。これらの製品を人間または動物に体内に導入する形態は、法律により厳格に禁止されています。これらのガイドラインに従うことは、研究と実験において法的および倫理的な基準の遵守を確実にするために重要です。