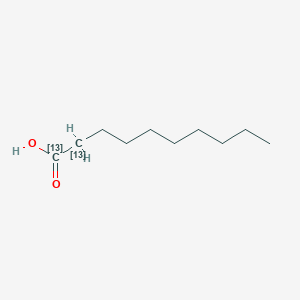
(1,2-13C2)decanoic acid
概要
説明
(1,2-¹³C₂)Decanoic acid is a stable isotope-labeled derivative of decanoic acid (C₁₀H₂₀O₂), a medium-chain fatty acid (MCFA) with 10 carbon atoms. The compound is specifically labeled with ¹³C isotopes at the first and second carbon positions (Figure 1). This labeling enables precise tracking in metabolic studies, isotopic dilution analysis, and tracer experiments in biological and environmental systems.
準備方法
Malonate Alkylation: Diethyl [1,2-¹³C₂]Malonate Route
Reaction Mechanism and Steps
The malonate alkylation method leverages the nucleophilic substitution of diethyl [1,2-¹³C₂]malonate with 1-bromooctane under alkaline conditions . The process involves:
-
Alkylation : Diethyl [1,2-¹³C₂]malonate reacts with 1-bromooctane in the presence of sodium ethoxide, forming diethyl [1,2-¹³C₂]octylmalonate.
-
Saponification : Hydrolysis with aqueous potassium hydroxide yields the dicarboxylic acid intermediate.
-
Decarboxylation : Thermal decarboxylation at 150–180°C eliminates one carboxyl group, producing (1,2-¹³C₂)decanoic acid .
Yield and Isotopic Purity
Parameter | Value |
---|---|
Overall Yield | 58–62% |
Isotopic Enrichment | 99 atom% ¹³C |
Purity (GC-MS) | >99% |
This method’s key advantage lies in its ability to introduce dual ¹³C labels via the malonate precursor. The use of isotopically enriched diethyl malonate ensures minimal cross-contamination from unlabeled species .
Nitrile Hydrolysis: [1-¹³C]Decanenitrile Oxidation
Synthetic Pathway
This two-step approach begins with the nucleophilic substitution of 1-bromononane with potassium [¹³C]cyanide (K¹³CN), followed by nitrile oxidation :
-
Nitrile Formation : 1-Bromononane reacts with K¹³CN (90% isotopic enrichment) to form [1-¹³C]decanenitrile.
-
Acid Hydrolysis : Oxidative hydrolysis with hydrogen peroxide in acidic medium yields [1-¹³C]decanoic acid.
Limitations and Modifications
While this method achieves 90% ¹³C enrichment at the first carbon, introducing a second label requires additional steps. For dual labeling, researchers have coupled this approach with microbial β-oxidation systems, where [1-¹³C]decanoic acid undergoes enzymatic degradation to retain ¹³C at the second position. However, this hybrid method complicates purification and reduces overall yield to 35–40%.
Cadmium-Mediated Coupling: Labeled Alkylcadmium Reagents
Reaction Design
A less common but highly specific method involves the coupling of [1,2-¹³C₂]alkylcadmium chloride with a dioic acid half-ester :
-
Cadmium Reagent Preparation : [1,2-¹³C₂]Dodecylmagnesium bromide reacts with cadmium chloride to form [1,2-¹³C₂]dodecylcadmium chloride.
-
Coupling Reaction : The cadmium reagent reacts with the half acid chloride of hexanedioic acid methyl ester, forming a ketone intermediate.
-
Reduction and Hydrolysis : Catalytic hydrogenation reduces the ketone to the alkane, followed by ester hydrolysis to yield (1,2-¹³C₂)decanoic acid.
Performance Metrics
Parameter | Value |
---|---|
Overall Yield | 45–50% |
Isotopic Enrichment | 98 atom% ¹³C |
Byproducts | <2% branched isomers |
This method’s complexity limits its adoption, though it offers precise control over label positioning in longer-chain analogs .
Comparative Analysis of Synthetic Methods
Method | Starting Materials | Steps | Yield (%) | ¹³C Enrichment | Key Advantage |
---|---|---|---|---|---|
Malonate Alkylation | Diethyl [1,2-¹³C₂]malonate | 3 | 58–62 | 99 | High isotopic purity |
Nitrile Hydrolysis | 1-Bromononane, K¹³CN | 2 | 70–75 | 90 (C1 only) | Simplicity |
Cadmium Coupling | [1,2-¹³C₂]Dodecylcadmium chloride | 4 | 45–50 | 98 | Positional specificity |
Purification and Quality Control
Post-synthesis purification typically involves:
-
Distillation : Fractional distillation under reduced pressure (0.1–0.5 mmHg) to isolate the labeled acid from unreacted precursors .
-
Crystallization : Recrystallization from ethanol-water mixtures enhances purity to >99.5% .
-
Chromatographic Validation : Gas chromatography-mass spectrometry (GC-MS) with a DB-5MS column confirms isotopic integrity, showing characteristic fragments at m/z 173 (M⁺–COO⁻) and 129 (M⁺–C₂H₄COO⁻). Nuclear magnetic resonance (¹³C NMR) further verifies label placement, with δ 34.2 ppm (C1) and δ 25.1 ppm (C2).
Industrial-Scale Production Considerations
While laboratory methods prioritize precision, industrial synthesis optimizes cost and throughput:
化学反応の分析
Core Reaction Types
(1,2-¹³C₂)Decanoic acid participates in three primary reaction categories:
β-Oxidation in Microbial Systems
-
In Pseudomonas aeruginosa cultures, (1,2-¹³C₂)decanoic acid undergoes β-oxidation to produce 3-oxodecanoic-1,2-¹³C₂ acid , with subsequent decarboxylation yielding acetyl-CoA fragments retaining ¹³C labels .
-
Isotopic tracing confirmed ~75% retention of ¹³C labels in downstream metabolites, validating its utility in tracking fatty acid degradation .
Fumarate Addition in Anaerobic Degradation
-
Under sulfate-reducing conditions, (1,2-¹³C₂)decanoic acid participates in fumarate addition reactions, forming 4-methyloctadecanoic acid-1,2-¹³C₂ as a terminal metabolite .
Isotopic Labeling in PFAS Analysis
-
Used as an internal standard in EPA Method 533 for quantifying perfluorodecanoic acid (PFDA) in environmental samples .
Stability and Degradation Byproducts
Condition | Outcome | Mechanism |
---|---|---|
Prolonged Acid Exposure | Decarboxylation to nonanoic acid-¹³C₂ | Loss of CO₂ from the carboxyl group |
UV Irradiation | Cleavage at C1-C2 bond | Radical-mediated fragmentation |
Comparative Reactivity with Analogues
Compound | Key Reaction Difference |
---|---|
Decanoic acid-10-¹³C | No ¹³C retention in β-oxidation products |
Hexanoic acid-1,2-¹³C₂ | Faster esterification kinetics |
Octanoic-d₁₅ acid | Deuterium exchange alters redox behavior |
Analytical Detection Data
Technique | Characteristic Signal |
---|---|
GC-MS (EI Mode) | m/z 173 → 129 (base peak, ¹³C₂ loss) |
NMR (¹³C) | δ 34.2 ppm (C1), δ 25.1 ppm (C2) |
科学的研究の応用
Metabolic Studies
Stable Isotope Tracing
(1,2-13C2)decanoic acid is primarily used in metabolic studies as a tracer to investigate lipid metabolism. Its incorporation into metabolic pathways allows researchers to track the fate of fatty acids in biological systems. Studies have shown that labeled fatty acids can help elucidate the mechanisms of energy metabolism and the role of specific fatty acids in health and disease.
- Example Case Study : A study utilized this compound to trace lipid metabolism in human subjects. The results indicated altered metabolic pathways in individuals with metabolic syndrome, highlighting the potential for targeted nutritional interventions .
Environmental Monitoring
Detection of Perfluoroalkyl Substances (PFAS)
The compound is also relevant in environmental science, particularly for the detection and quantification of perfluoroalkyl substances (PFAS). The isotopic labeling aids in improving the sensitivity and specificity of analytical methods used to monitor these contaminants in various matrices such as water, soil, and biological samples.
- Analytical Methodology : In a study examining PFAS contamination, this compound was employed as an internal standard during liquid chromatography-tandem mass spectrometry (LC-MS/MS) analysis. This approach enhanced the accuracy of quantifying trace levels of PFAS in environmental samples .
Analytical Chemistry
Isotope Dilution Mass Spectrometry (IDMS)
this compound is frequently used in IDMS for quantitative analysis of fatty acids and related compounds. Its stable isotope nature allows for precise calibration of analytical methods, ensuring reliable and reproducible results.
- Application Example : A comprehensive evaluation of PFAS removal from contaminated water sources demonstrated the effectiveness of using this compound as a standard in IDMS. The method provided robust data on the removal efficiencies of various treatment processes .
Data Tables
Application Area | Methodology | Key Findings/Outcomes |
---|---|---|
Metabolic Studies | Stable Isotope Tracing | Altered lipid metabolism in metabolic syndrome |
Environmental Monitoring | LC-MS/MS with Isotope Labeling | Enhanced detection of PFAS contaminants |
Analytical Chemistry | IDMS for Quantitative Analysis | Improved accuracy in quantifying trace levels |
作用機序
(1,2-13C2)decanoic acid exerts its effects primarily through its role in lipid metabolism and energy production. It has been shown to improve mitochondrial function and energy metabolism in neuronal cell models and fibroblasts from patients with mitochondrial disorders . The compound influences the activity of sirtuins, a group of NAD-dependent enzymes that regulate energy metabolism, leading to enhanced mitochondrial respiratory chain function .
類似化合物との比較
Key Characteristics:
- Molecular Formula : C₁₀H₂₀O₂ (¹³C₂ substitution at C1 and C2).
- Applications: Biological Studies: Used to investigate fatty acid metabolism in Pseudomonas aeruginosa for tracking 4-hydroxy-2-alkylquinoline (HAQ) biosynthesis . Analytical Chemistry: Serves as a surrogate or internal standard in liquid chromatography-tandem mass spectrometry (LC-MS/MS) for quantifying perfluoroalkyl acids (PFAAs) in environmental samples . Metabolic Tracing: Monitors β-oxidation pathways due to its labeled terminal carbons, critical in studies of lipid metabolism .
Isotope-Labeled Decanoic Acid Derivatives
Table 1: Comparison of Isotopologues of Decanoic Acid
Notes:
- ¹³C vs. D Labeling : ¹³C-labeled compounds avoid chromatographic retention time shifts observed with deuterated analogs, improving accuracy in LC-MS/MS .
- Positional Specificity: (1,2-¹³C₂)Decanoic acid is ideal for tracking β-oxidation, whereas terminal deuteration (e.g., C9–C10) is used for studying elongation or degradation pathways .
Perfluorinated Analogs
Table 2: Comparison with Perfluoroalkyl Carboxylic Acids (PFCAs)
Notes:
- Structural Differences: MPFDA is fully fluorinated and ¹³C-labeled, whereas (1,2-¹³C₂)decanoic acid retains hydrogen atoms. Fluorination increases molecular weight and hydrophobicity (logKOW = 5.48) .
- Analytical Utility : MPFDA is used as a mass spectrometry internal standard to distinguish from unlabeled PFDA via precursor ion mass shifts (515.17 vs. 513.17) .
Chain-Length Variants of ¹³C-Labeled Fatty Acids
Table 3: Comparison with Other ¹³C-Labeled Fatty Acids
Notes:
- Chain-Length Effects: Medium-chain (C10) vs. long-chain (C12) fatty acids exhibit differences in solubility and metabolic pathways. Decanoic acid is preferentially β-oxidized in mitochondria, while dodecanoic acid requires carnitine shuttle transport .
- Positional Labeling: C1–C2 labeling in decanoic acid targets initial β-oxidation steps, whereas single-position labels (e.g., C2 in dodecanoic acid) track specific enzymatic modifications .
Environmental Analysis
生物活性
(1,2-13C2)decanoic acid is a stable isotope-labeled fatty acid that has garnered attention for its potential biological activities and applications in various fields, including microbiology, pharmacology, and metabolic studies. This article explores the biological activity of this compound, highlighting its mechanisms of action, effects on microbial metabolism, and implications for drug development.
Chemical Structure and Properties
This compound is a medium-chain fatty acid with a carbon chain length of ten carbons. The incorporation of stable isotopes (13C) allows for tracking metabolic pathways in biological systems. Its structure can be represented as follows:
Microbial Metabolism
Research has demonstrated that this compound can influence microbial metabolism significantly. In a study involving Pseudomonas aeruginosa, the addition of 1 mM this compound to cultures led to increased production of 3-oxodecanoic acid through β-oxidation. This metabolic pathway is crucial for the synthesis of signaling molecules such as hydroxyalkylquinolines (HAQs), which play a role in bacterial virulence and communication .
Table 1: Effects of this compound on Microbial Metabolism
Parameter | Control Group | This compound Group |
---|---|---|
Production of 3-Oxodecanoic Acid | Baseline | Increased |
HAQ Synthesis | Low | Elevated |
Pyocyanin Production | Low | Increased |
The increase in pyocyanin production indicates that this compound may enhance the pathogenic potential of Pseudomonas aeruginosa by modulating its metabolic pathways .
Pharmacological Implications
The biological activity of this compound extends to pharmacology. Its role as a fatty acid suggests potential therapeutic applications due to its effects on lipid metabolism and cell signaling. Fatty acids have been studied for their anti-inflammatory properties and ability to modulate immune responses .
Case Studies
Case Study 1: Antimicrobial Activity
In vitro studies have shown that medium-chain fatty acids like decanoic acid exhibit antimicrobial properties against various pathogens. The incorporation of the stable isotope label allows researchers to trace the metabolic pathways involved in these effects. For instance, decanoic acid has been found to disrupt microbial membranes and inhibit growth in certain bacterial strains.
Case Study 2: Metabolic Tracking
A study utilizing this compound in metabolic tracking demonstrated its utility in understanding lipid metabolism in human subjects. Participants consuming labeled decanoic acid showed distinct metabolic profiles when analyzed through mass spectrometry, providing insights into how dietary fats are processed by the body .
Q & A
Basic Research Questions
Q. How is (1,2-<sup>13</sup>C2)decanoic acid synthesized for isotopic labeling in metabolic studies?
The synthesis typically involves introducing <sup>13</sup>C isotopes at specific carbon positions via precursor-directed biosynthesis or chemical synthesis. For example, tetradecanoic-1,2-<sup>13</sup>C2 acid (a structurally similar compound) is synthesized using isotopically labeled starting materials like <sup>13</sup>C-enriched acetic acid or malonyl-CoA derivatives, which are enzymatically elongated during fatty acid biosynthesis . This method ensures high isotopic purity (>99 atom% <sup>13</sup>C) critical for tracing metabolic pathways.
Q. What analytical techniques are optimized for quantifying (1,2-<sup>13</sup>C2)decanoic acid in environmental samples?
Liquid chromatography-tandem mass spectrometry (LC-MS/MS) is the gold standard, utilizing transitions like m/z 515.2 → 470.1 for the labeled compound and m/z 512.9 → 468.9 for the unlabeled analog. This allows precise differentiation and quantification in complex matrices such as water or biological fluids . Isotopic separation is achieved using C18 columns with mobile phases containing ammonium acetate and methanol.
Q. Why is (1,2-<sup>13</sup>C2)decanoic acid used as an internal standard in PFAS analysis?
The compound serves as a stable isotope-labeled surrogate to correct for matrix effects and instrument variability during PFAS quantification. For example, in EPA Method 537.1, it compensates for losses during solid-phase extraction (SPE) and improves accuracy in detecting perfluorodecanoic acid (PFDA) . Its structural similarity to target analytes ensures comparable extraction recovery and ionization efficiency.
Q. How does isotopic labeling enhance studies on fatty acid metabolism in microbial systems?
By incorporating (1,2-<sup>13</sup>C2)decanoic acid into microbial cultures (e.g., Pseudomonas aeruginosa), researchers track its incorporation into metabolites like 4-hydroxy-2-alkylquinolines (HAQs) using <sup>13</sup>C-NMR or mass spectrometry. This reveals carbon flux through β-oxidation pathways and biosynthetic routes, as demonstrated in studies where labeled decanoic acid increased HAQ production .
Q. What precautions are necessary when handling (1,2-<sup>13</sup>C2)decanoic acid in cell-based assays?
Key precautions include:
- Using solvent-resistant containers to avoid leaching of interfering compounds (e.g., PFAS from PTFE tubes).
- Validating cell membrane permeability via electrochemical impedance spectroscopy to ensure uptake without disrupting lipid bilayers .
- Confirming isotopic stability under experimental conditions (e.g., pH, temperature) to prevent <sup>13</sup>C loss.
Advanced Research Questions
Q. How can researchers resolve low surrogate recovery rates of (1,2-<sup>13</sup>C2)decanoic acid in PFAS analysis?
Low recovery (< QC limits) often stems from matrix interference or SPE cartridge inefficiency. Mitigation strategies include:
- Optimizing SPE sorbents (e.g., switching from C18 to S-DVB cartridges to reduce PFAS contamination) .
- Adjusting spiking concentrations to account for competitive binding in high-organic matrices.
- Validating recovery using alternative surrogates (e.g., <sup>13</sup>C4-PFOS) to identify systemic biases .
Q. What experimental design considerations are critical when using (1,2-<sup>13</sup>C2)decanoic acid to study bacterial quorum sensing?
Key considerations include:
- Dose optimization : Excess decanoic acid inhibits bacterial growth, necessitating dose-response assays (e.g., 0.1–1 mM tested in P. aeruginosa PA14 cultures) .
- Isotopic dilution controls : Unlabeled decanoic acid controls must be included to distinguish isotopic enrichment from natural abundance.
- Metabolite extraction timing : Sampling at mid-log phase ensures maximal HAQ production without degradation.
Q. How can cross-contamination between isotopic and native decanoic acid be minimized in mass spectrometry?
Cross-contamination arises from overlapping isotopic peaks or carryover. Solutions include:
- Employing high-resolution mass spectrometers (HRMS) to resolve <sup>13</sup>C2 (Δm/z = +2.02) from native ions.
- Implementing stringent column wash protocols (e.g., 90% methanol/10% acetone for 10 min post-run) .
- Using separate LC systems for labeled and unlabeled analytes.
Q. What statistical methods address variability in (1,2-<sup>13</sup>C2)decanoic acid tracer studies?
Isotope ratio mass spectrometry (IRMS) data require correction for natural <sup>13</sup>C abundance and kinetic isotope effects. Bayesian hierarchical models or Monte Carlo simulations are used to propagate uncertainty in metabolic flux analysis . Additionally, surrogate-normalized quantification (e.g., using <sup>13</sup>C2-PFDA as an internal standard) reduces technical variability in environmental samples .
Q. How does (1,2-<sup>13</sup>C2)decanoic acid improve reproducibility in neurochemical studies?
In ketogenic diet (KD) research, the compound enables precise measurement of brain uptake and β-oxidation rates. Methodological steps include:
特性
IUPAC Name |
(1,2-13C2)decanoic acid | |
---|---|---|
Source | PubChem | |
URL | https://pubchem.ncbi.nlm.nih.gov | |
Description | Data deposited in or computed by PubChem | |
InChI |
InChI=1S/C10H20O2/c1-2-3-4-5-6-7-8-9-10(11)12/h2-9H2,1H3,(H,11,12)/i9+1,10+1 | |
Source | PubChem | |
URL | https://pubchem.ncbi.nlm.nih.gov | |
Description | Data deposited in or computed by PubChem | |
InChI Key |
GHVNFZFCNZKVNT-OJJJIBSVSA-N | |
Source | PubChem | |
URL | https://pubchem.ncbi.nlm.nih.gov | |
Description | Data deposited in or computed by PubChem | |
Canonical SMILES |
CCCCCCCCCC(=O)O | |
Source | PubChem | |
URL | https://pubchem.ncbi.nlm.nih.gov | |
Description | Data deposited in or computed by PubChem | |
Isomeric SMILES |
CCCCCCCC[13CH2][13C](=O)O | |
Source | PubChem | |
URL | https://pubchem.ncbi.nlm.nih.gov | |
Description | Data deposited in or computed by PubChem | |
Molecular Formula |
C10H20O2 | |
Source | PubChem | |
URL | https://pubchem.ncbi.nlm.nih.gov | |
Description | Data deposited in or computed by PubChem | |
DSSTOX Substance ID |
DTXSID00584355 | |
Record name | (1,2-~13~C_2_)Decanoic acid | |
Source | EPA DSSTox | |
URL | https://comptox.epa.gov/dashboard/DTXSID00584355 | |
Description | DSSTox provides a high quality public chemistry resource for supporting improved predictive toxicology. | |
Molecular Weight |
174.25 g/mol | |
Source | PubChem | |
URL | https://pubchem.ncbi.nlm.nih.gov | |
Description | Data deposited in or computed by PubChem | |
CAS No. |
287111-30-2 | |
Record name | (1,2-~13~C_2_)Decanoic acid | |
Source | EPA DSSTox | |
URL | https://comptox.epa.gov/dashboard/DTXSID00584355 | |
Description | DSSTox provides a high quality public chemistry resource for supporting improved predictive toxicology. | |
Record name | 287111-30-2 | |
Source | European Chemicals Agency (ECHA) | |
URL | https://echa.europa.eu/information-on-chemicals | |
Description | The European Chemicals Agency (ECHA) is an agency of the European Union which is the driving force among regulatory authorities in implementing the EU's groundbreaking chemicals legislation for the benefit of human health and the environment as well as for innovation and competitiveness. | |
Explanation | Use of the information, documents and data from the ECHA website is subject to the terms and conditions of this Legal Notice, and subject to other binding limitations provided for under applicable law, the information, documents and data made available on the ECHA website may be reproduced, distributed and/or used, totally or in part, for non-commercial purposes provided that ECHA is acknowledged as the source: "Source: European Chemicals Agency, http://echa.europa.eu/". Such acknowledgement must be included in each copy of the material. ECHA permits and encourages organisations and individuals to create links to the ECHA website under the following cumulative conditions: Links can only be made to webpages that provide a link to the Legal Notice page. | |
Synthesis routes and methods
Procedure details
試験管内研究製品の免責事項と情報
BenchChemで提示されるすべての記事および製品情報は、情報提供を目的としています。BenchChemで購入可能な製品は、生体外研究のために特別に設計されています。生体外研究は、ラテン語の "in glass" に由来し、生物体の外で行われる実験を指します。これらの製品は医薬品または薬として分類されておらず、FDAから任何の医療状態、病気、または疾患の予防、治療、または治癒のために承認されていません。これらの製品を人間または動物に体内に導入する形態は、法律により厳格に禁止されています。これらのガイドラインに従うことは、研究と実験において法的および倫理的な基準の遵守を確実にするために重要です。