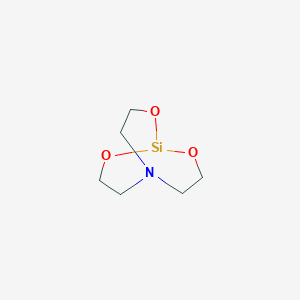
シラトラン
概要
説明
Silatrane is a unique organosilicon compound characterized by its tricyclic structure, which includes a silicon atom coordinated to a nitrogen atom through an intramolecular bond. This distinctive structure imparts silatrane with remarkable stability and reactivity, making it a subject of extensive research in various scientific fields .
科学的研究の応用
Silatrane has a wide range of applications in scientific research, including:
Chemistry: Used as a precursor for advanced polymer materials and silicon-based catalysts.
Biology: Exhibits biological activity, including antibacterial, antifungal, and anticancer properties.
Medicine: Potential drug candidates due to their high physiological activity.
Industry: Employed in surface modification, plant growth biostimulants, and as components in high-tech devices.
作用機序
Target of Action
Silatrane is a unique compound that has been found to interact with a variety of biological targets. Some of the primary targets of Silatrane include proteins and nucleic acids . The compound has been found to stimulate the biosynthesis of these crucial biomolecules, indicating its potential role in cellular growth and development .
Mode of Action
The mode of action of Silatrane is largely attributed to its unique structure, which includes a variation of Si–N bond length based on the axial substituent of Si . This structural feature allows Silatrane to interact with its targets in a specific manner, leading to changes in their activity. For instance, Silatrane has been found to regulate the activity of enzymes and hormones , which play crucial roles in various biological processes.
Biochemical Pathways
Silatrane affects several biochemical pathways due to its interaction with proteins and nucleic acids. By stimulating the biosynthesis of these biomolecules, Silatrane can influence the pathways they are involved in, leading to downstream effects. For example, the stimulation of protein synthesis can impact pathways related to cellular growth and development .
Pharmacokinetics
Its high physiological activity suggests that it may have good bioavailability
Result of Action
The action of Silatrane results in a variety of molecular and cellular effects. It has been found to exert a protective effect during hypo- and hyperthermia, oxygen starvation, and radiation damage . Additionally, Silatrane has shown potential for medical use to heal wounds or stimulate hair growth . It also exhibits pharmacological properties such as antitumor, anticancer, antibacterial, anti-inflammatory, and fungicidal activity .
Action Environment
The action of Silatrane can be influenced by environmental factors. For instance, Silatrane has the ability to mildly control hydrolysis, allowing it to form extremely stable and smooth siloxane monolayers almost on any surface . This suggests that the compound’s action, efficacy, and stability may be influenced by the presence of water and other environmental conditions.
生化学分析
Biochemical Properties
Silatrane’s biochemical properties are determined by its basicity, which depends on its stereoelectronic structure . It interacts with various biomolecules, and its reactivity and physicochemical properties are influenced by these interactions .
Cellular Effects
The specific biological activity of Silatrane has attracted significant attention . It influences cell function by interacting with various cellular processes, including cell signaling pathways, gene expression, and cellular metabolism .
Molecular Mechanism
Silatrane exerts its effects at the molecular level through binding interactions with biomolecules, enzyme inhibition or activation, and changes in gene expression . Its unique reactivity is due to both the electron density distribution and the spatial structure .
Temporal Effects in Laboratory Settings
The effects of Silatrane change over time in laboratory settings . Information on the product’s stability, degradation, and any long-term effects on cellular function observed in in vitro or in vivo studies is currently being researched .
Dosage Effects in Animal Models
The effects of Silatrane vary with different dosages in animal models . Studies are ongoing to determine any threshold effects, as well as any toxic or adverse effects at high doses .
Metabolic Pathways
Silatrane is involved in various metabolic pathways . It interacts with enzymes and cofactors, and can affect metabolic flux or metabolite levels .
Transport and Distribution
The transport and distribution of Silatrane within cells and tissues is a complex process that involves interactions with transporters and binding proteins . Research is ongoing to understand its effects on localization or accumulation .
Subcellular Localization
The subcellular localization of Silatrane and its effects on activity or function are areas of active research . This includes any targeting signals or post-translational modifications that direct it to specific compartments or organelles .
準備方法
Synthetic Routes and Reaction Conditions: Silatrane can be synthesized through several methods, with one of the most common being the transesterification of trialkoxysilanes with triethanolamine or triisopropanolamine. This reaction typically occurs under mild conditions and can be catalyzed by various organocatalysts, such as amidine derivatives . Another method involves the boratrane approach, which utilizes boron-containing intermediates to facilitate the formation of the silatrane structure .
Industrial Production Methods: In industrial settings, the production of silatrane often involves the use of scalable and efficient protocols. For instance, a solvent-free organocatalytic approach has been developed, which allows for the large-scale synthesis of silatrane derivatives with high yields and minimal environmental impact .
化学反応の分析
Types of Reactions: Silatrane undergoes various chemical reactions, including:
Oxidation: Silatrane can be oxidized to form siloxane derivatives.
Reduction: Reduction reactions can convert silatrane into simpler organosilicon compounds.
Substitution: Silatrane can participate in nucleophilic substitution reactions, although these occur slowly due to the strong chelating effect of the silatrane cage
Common Reagents and Conditions:
Oxidation: Common oxidizing agents include hydrogen peroxide and peracids.
Reduction: Reducing agents such as lithium aluminum hydride can be used.
Substitution: Nucleophiles like amines and alcohols can react with silatrane under appropriate conditions.
Major Products:
Oxidation: Siloxane derivatives.
Reduction: Simpler organosilicon compounds.
Substitution: Various substituted silatranes depending on the nucleophile used.
類似化合物との比較
3-Aminopropylsilatrane: A derivative with similar biological activity and applications.
1-Halo-3,7,10-trimethylsilatrane: Exhibits higher basicity compared to other silatranes.
Uniqueness: Silatrane’s unique tricyclic structure and strong intramolecular coordination bond distinguish it from other organosilicon compounds. This structure imparts exceptional stability and reactivity, making silatrane a versatile compound in various scientific and industrial applications .
特性
IUPAC Name |
2,8,9-trioxa-5-aza-1-silabicyclo[3.3.3]undecane | |
---|---|---|
Details | Computed by Lexichem TK 2.7.0 (PubChem release 2021.10.14) | |
Source | PubChem | |
URL | https://pubchem.ncbi.nlm.nih.gov | |
Description | Data deposited in or computed by PubChem | |
InChI |
InChI=1S/C6H13NO3Si/c1-4-8-11-9-5-2-7(1)3-6-10-11/h11H,1-6H2 | |
Details | Computed by InChI 1.0.6 (PubChem release 2021.10.14) | |
Source | PubChem | |
URL | https://pubchem.ncbi.nlm.nih.gov | |
Description | Data deposited in or computed by PubChem | |
InChI Key |
PZRZYNDNPQRQRN-UHFFFAOYSA-N | |
Details | Computed by InChI 1.0.6 (PubChem release 2021.10.14) | |
Source | PubChem | |
URL | https://pubchem.ncbi.nlm.nih.gov | |
Description | Data deposited in or computed by PubChem | |
Canonical SMILES |
C1CO[SiH]2OCCN1CCO2 | |
Details | Computed by OEChem 2.3.0 (PubChem release 2021.10.14) | |
Source | PubChem | |
URL | https://pubchem.ncbi.nlm.nih.gov | |
Description | Data deposited in or computed by PubChem | |
Molecular Formula |
C6H13NO3Si | |
Details | Computed by PubChem 2.2 (PubChem release 2021.10.14) | |
Source | PubChem | |
URL | https://pubchem.ncbi.nlm.nih.gov | |
Description | Data deposited in or computed by PubChem | |
Molecular Weight |
175.26 g/mol | |
Details | Computed by PubChem 2.2 (PubChem release 2021.10.14) | |
Source | PubChem | |
URL | https://pubchem.ncbi.nlm.nih.gov | |
Description | Data deposited in or computed by PubChem | |
CAS No. |
283-60-3 | |
Record name | Silatrane | |
Source | CAS Common Chemistry | |
URL | https://commonchemistry.cas.org/detail?cas_rn=283-60-3 | |
Description | CAS Common Chemistry is an open community resource for accessing chemical information. Nearly 500,000 chemical substances from CAS REGISTRY cover areas of community interest, including common and frequently regulated chemicals, and those relevant to high school and undergraduate chemistry classes. This chemical information, curated by our expert scientists, is provided in alignment with our mission as a division of the American Chemical Society. | |
Explanation | The data from CAS Common Chemistry is provided under a CC-BY-NC 4.0 license, unless otherwise stated. | |
Retrosynthesis Analysis
AI-Powered Synthesis Planning: Our tool employs the Template_relevance Pistachio, Template_relevance Bkms_metabolic, Template_relevance Pistachio_ringbreaker, Template_relevance Reaxys, Template_relevance Reaxys_biocatalysis model, leveraging a vast database of chemical reactions to predict feasible synthetic routes.
One-Step Synthesis Focus: Specifically designed for one-step synthesis, it provides concise and direct routes for your target compounds, streamlining the synthesis process.
Accurate Predictions: Utilizing the extensive PISTACHIO, BKMS_METABOLIC, PISTACHIO_RINGBREAKER, REAXYS, REAXYS_BIOCATALYSIS database, our tool offers high-accuracy predictions, reflecting the latest in chemical research and data.
Strategy Settings
Precursor scoring | Relevance Heuristic |
---|---|
Min. plausibility | 0.01 |
Model | Template_relevance |
Template Set | Pistachio/Bkms_metabolic/Pistachio_ringbreaker/Reaxys/Reaxys_biocatalysis |
Top-N result to add to graph | 6 |
Feasible Synthetic Routes
試験管内研究製品の免責事項と情報
BenchChemで提示されるすべての記事および製品情報は、情報提供を目的としています。BenchChemで購入可能な製品は、生体外研究のために特別に設計されています。生体外研究は、ラテン語の "in glass" に由来し、生物体の外で行われる実験を指します。これらの製品は医薬品または薬として分類されておらず、FDAから任何の医療状態、病気、または疾患の予防、治療、または治癒のために承認されていません。これらの製品を人間または動物に体内に導入する形態は、法律により厳格に禁止されています。これらのガイドラインに従うことは、研究と実験において法的および倫理的な基準の遵守を確実にするために重要です。