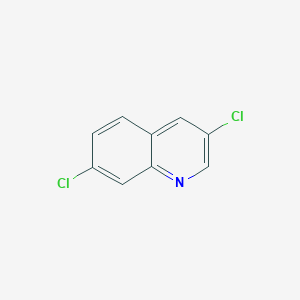
3,7-Dichloroquinoline
概要
説明
3,7-Dichloroquinoline is a two-ring heterocyclic compound . It is used as a chemical intermediate to aminoquinoline antimalarial drugs including amodiaquine, chloroquine, and hydroxychloroquine . It is also used as a drug chloroquine phosphate intermediate .
Molecular Structure Analysis
The molecular structure of 3,7-Dichloroquinoline has been studied using various techniques. The crystal structure is stabilized by aromatic π-π stacking between the benzene and the pyridine rings of neighboring molecules . In addition, weak inter-molecular C-H⋯N hydrogen bonds are present in the structure .
Physical And Chemical Properties Analysis
The physical and chemical properties of 3,7-Dichloroquinoline can be found in various databases . The molecular weight of 3,7-Dichloroquinoline is 198.05 g/mol . It has a XLogP3-AA value of 3.4, indicating its lipophilicity . The compound has no hydrogen bond donors and one hydrogen bond acceptor .
科学的研究の応用
Antimalarial Activity
3,7-Dichloroquinoline derivatives have shown significant promise in the fight against malaria. They exhibit potent in-vitro antiplasmodial efficacy , inhibiting the growth of sensitive strains of Plasmodium falciparum with IC50 values indicating high potency . This suggests that these compounds could be developed into effective antimalarial agents, providing a new avenue for combating this life-threatening disease.
Vector Control for Mosquito-Borne Diseases
The larvicidal and pupicidal properties of 3,7-Dichloroquinoline derivatives make them excellent candidates for controlling mosquito populations, particularly those that serve as vectors for malaria and dengue . By targeting the larval and pupal stages of mosquitoes, these compounds can help reduce the incidence of these diseases.
Antibacterial Applications
Research has demonstrated that 3,7-Dichloroquinoline and its analogs possess antibacterial properties. They have been tested against various bacterial strains such as Staphylococcus aureus, Escherichia coli, Pseudomonas aeruginosa, and Streptococcus pyogenes, showing good activity and potential as antibacterial agents .
Antioxidant Properties
Some novel chloroquinoline analogs, including derivatives of 3,7-Dichloroquinoline, have been found to exhibit strong antioxidant activity. This is measured using the DPPH radical scavenging assay, where these compounds have shown IC50 values comparable to ascorbic acid, indicating their potential as antioxidants .
Herbicidal and Fungicidal Activity
The structural analogs of 3,7-Dichloroquinoline have been explored for their use as herbicides and fungicides. They display excellent control efficiency against weeds like Echinochloa crusgalli and show good in-vitro activity against fungal pathogens such as Phytophthora capsici, Phytophthora sojae, and Phytophthora infestans . This highlights their potential in agricultural applications to protect crops from weeds and fungal diseases.
Anticancer Research
Quinoline derivatives are known for their anticancer properties, and 3,7-Dichloroquinoline is no exception. It serves as a scaffold for developing bioactive molecules that can be used in anticancer research. The ability of these compounds to inhibit the growth of cancer cells makes them valuable in the search for new cancer treatments .
Antiviral Efficacy
The derivatives of 3,7-Dichloroquinoline have shown activity against viral pathogens, including serotype 2 of the dengue virus (DENV-2). This suggests that these compounds could be further researched for their potential as antiviral agents .
Molecular Docking Studies
Molecular docking studies of 3,7-Dichloroquinoline analogs have revealed their binding patterns with important biological targets such as topoisomerase II β and E. coli DNA gyrase B. These studies are crucial for understanding the mode of action of these compounds and for the development of drugs with specific target interactions .
Safety and Hazards
When handling 3,7-Dichloroquinoline, it is recommended to wear personal protective equipment, ensure adequate ventilation, and avoid getting the compound in eyes, on skin, or on clothing . Ingestion and inhalation should also be avoided . The compound should be kept in a dry, cool, and well-ventilated place .
作用機序
Target of Action
3,7-Dichloroquinoline is a two-ring heterocyclic compound used as a chemical intermediate to aminoquinoline antimalarial drugs including amodiaquine, chloroquine, and hydroxychloroquine . It is also used in the synthesis of potential novel pesticide compounds . The primary targets of 3,7-Dichloroquinoline are the Plasmodium species, which cause malaria, and certain agricultural pests .
Mode of Action
The mode of action of 3,7-Dichloroquinoline is similar to that of chloroquine, which inhibits heme detoxification within the Plasmodium food vacuole, leading to the accumulation of toxic heme and parasite death . In the context of its pesticidal activity, it has been shown to have excellent control efficiency against Echinochloa crusgalli, a type of weed, and also showed good fungicidal in vitro activity against Phytophthora capsici, Phytophthora sojae, and Phytophthora infestans .
Biochemical Pathways
The biochemical pathways affected by 3,7-Dichloroquinoline are primarily those involved in the life cycle of the Plasmodium species and the growth of certain agricultural pests . The compound interferes with the detoxification of heme within the Plasmodium food vacuole, disrupting the parasite’s survival .
Pharmacokinetics
It is known that related compounds like chloroquine are rapidly absorbed from the gut . The ADME (Absorption, Distribution, Metabolism, and Excretion) properties of 3,7-Dichloroquinoline and their impact on bioavailability would need further investigation.
Result of Action
The result of 3,7-Dichloroquinoline’s action is the death of the Plasmodium species and certain agricultural pests. It causes significant growth inhibition of both sensitive strains of Plasmodium falciparum . In terms of its pesticidal activity, it has been shown to control the growth of Echinochloa crusgalli effectively .
Action Environment
The action of 3,7-Dichloroquinoline can be influenced by environmental factors. For instance, the effectiveness of its pesticidal activity may vary depending on the specific conditions of the agricultural environment
特性
IUPAC Name |
3,7-dichloroquinoline | |
---|---|---|
Source | PubChem | |
URL | https://pubchem.ncbi.nlm.nih.gov | |
Description | Data deposited in or computed by PubChem | |
InChI |
InChI=1S/C9H5Cl2N/c10-7-2-1-6-3-8(11)5-12-9(6)4-7/h1-5H | |
Source | PubChem | |
URL | https://pubchem.ncbi.nlm.nih.gov | |
Description | Data deposited in or computed by PubChem | |
InChI Key |
SLJNJMNYSNPHJE-UHFFFAOYSA-N | |
Source | PubChem | |
URL | https://pubchem.ncbi.nlm.nih.gov | |
Description | Data deposited in or computed by PubChem | |
Canonical SMILES |
C1=CC(=CC2=NC=C(C=C21)Cl)Cl | |
Source | PubChem | |
URL | https://pubchem.ncbi.nlm.nih.gov | |
Description | Data deposited in or computed by PubChem | |
Molecular Formula |
C9H5Cl2N | |
Source | PubChem | |
URL | https://pubchem.ncbi.nlm.nih.gov | |
Description | Data deposited in or computed by PubChem | |
DSSTOX Substance ID |
DTXSID50593376 | |
Record name | 3,7-Dichloroquinoline | |
Source | EPA DSSTox | |
URL | https://comptox.epa.gov/dashboard/DTXSID50593376 | |
Description | DSSTox provides a high quality public chemistry resource for supporting improved predictive toxicology. | |
Molecular Weight |
198.05 g/mol | |
Source | PubChem | |
URL | https://pubchem.ncbi.nlm.nih.gov | |
Description | Data deposited in or computed by PubChem | |
Product Name |
3,7-Dichloroquinoline | |
CAS RN |
152210-25-8 | |
Record name | 3,7-Dichloroquinoline | |
Source | CAS Common Chemistry | |
URL | https://commonchemistry.cas.org/detail?cas_rn=152210-25-8 | |
Description | CAS Common Chemistry is an open community resource for accessing chemical information. Nearly 500,000 chemical substances from CAS REGISTRY cover areas of community interest, including common and frequently regulated chemicals, and those relevant to high school and undergraduate chemistry classes. This chemical information, curated by our expert scientists, is provided in alignment with our mission as a division of the American Chemical Society. | |
Explanation | The data from CAS Common Chemistry is provided under a CC-BY-NC 4.0 license, unless otherwise stated. | |
Record name | 3,7-Dichloroquinoline | |
Source | EPA DSSTox | |
URL | https://comptox.epa.gov/dashboard/DTXSID50593376 | |
Description | DSSTox provides a high quality public chemistry resource for supporting improved predictive toxicology. | |
Record name | Quinoline, 3,7-dichloro | |
Source | European Chemicals Agency (ECHA) | |
URL | https://echa.europa.eu/information-on-chemicals | |
Description | The European Chemicals Agency (ECHA) is an agency of the European Union which is the driving force among regulatory authorities in implementing the EU's groundbreaking chemicals legislation for the benefit of human health and the environment as well as for innovation and competitiveness. | |
Explanation | Use of the information, documents and data from the ECHA website is subject to the terms and conditions of this Legal Notice, and subject to other binding limitations provided for under applicable law, the information, documents and data made available on the ECHA website may be reproduced, distributed and/or used, totally or in part, for non-commercial purposes provided that ECHA is acknowledged as the source: "Source: European Chemicals Agency, http://echa.europa.eu/". Such acknowledgement must be included in each copy of the material. ECHA permits and encourages organisations and individuals to create links to the ECHA website under the following cumulative conditions: Links can only be made to webpages that provide a link to the Legal Notice page. | |
Retrosynthesis Analysis
AI-Powered Synthesis Planning: Our tool employs the Template_relevance Pistachio, Template_relevance Bkms_metabolic, Template_relevance Pistachio_ringbreaker, Template_relevance Reaxys, Template_relevance Reaxys_biocatalysis model, leveraging a vast database of chemical reactions to predict feasible synthetic routes.
One-Step Synthesis Focus: Specifically designed for one-step synthesis, it provides concise and direct routes for your target compounds, streamlining the synthesis process.
Accurate Predictions: Utilizing the extensive PISTACHIO, BKMS_METABOLIC, PISTACHIO_RINGBREAKER, REAXYS, REAXYS_BIOCATALYSIS database, our tool offers high-accuracy predictions, reflecting the latest in chemical research and data.
Strategy Settings
Precursor scoring | Relevance Heuristic |
---|---|
Min. plausibility | 0.01 |
Model | Template_relevance |
Template Set | Pistachio/Bkms_metabolic/Pistachio_ringbreaker/Reaxys/Reaxys_biocatalysis |
Top-N result to add to graph | 6 |
Feasible Synthetic Routes
Q & A
Q1: What is 3,7-Dichloroquinoline-8-carboxylic acid primarily known for?
A1: 3,7-Dichloroquinoline-8-carboxylic acid, commercially known as quinclorac, is a herbicide widely used for controlling unwanted plant growth. [] It is particularly effective against leafy spurge, a noxious weed. []
Q2: How does quinclorac behave in a paddy field environment?
A2: Research using radiolabeled quinclorac in a simulated paddy field (lysimeter) revealed that it degrades slowly. Only 0.71% of the applied quinclorac was lost as carbon dioxide over 14 weeks. The study also indicated minimal leaching into lower soil layers, suggesting it primarily stays in the topsoil. []
Q3: Are there concerns about quinclorac affecting non-target species like the western prairie fringed orchid?
A4: Studies show that quinclorac applied at recommended rates in late season (after orchid senescence) did not negatively impact the orchid's survival, growth, or seed production. This suggests quinclorac could be a valuable tool for managing leafy spurge in sensitive habitats while minimizing harm to the orchid. []
Q4: How does the structure of quinclorac influence its interaction with soil?
A5: The adsorption of quinclorac and its analog, quinmerac, onto various clay minerals is strongly influenced by the clay's exchangeable cations. For example, quinclorac adsorption is significant onto iron-saturated clay but minimal onto calcium or sodium-saturated clays. This difference is attributed to the protonation of the quinoline nitrogen in acidic conditions near iron, facilitating adsorption. []
Q5: What are the structural features of 3,7-Dichloroquinoline derivatives?
A7: 3,7-Dichloroquinoline derivatives often feature various substituents (represented by "R" in research) attached to the core quinoline structure. These variations in "R" groups influence the compound's herbicidal activity. []
Q6: How does the structure of 3,7-dichloroquinoline-8-carboxylic acid influence its crystal structure?
A8: In its pure form, 3,7-dichloroquinoline-8-carboxylic acid molecules arrange themselves in the crystal lattice primarily through π-π stacking interactions between the aromatic rings and O—H⋯N hydrogen bonding. []
Q7: What happens when you react the Weinreb amide of 3,7-dichloroquinoline-8-carboxylic acid with organometallic reagents?
A9: Instead of yielding the expected ketone derivatives, reacting the Weinreb amide of 3,7-dichloroquinoline-8-carboxylic acid with Grignard or organolithium reagents predominantly produces 2-substituted derivatives. This unexpected outcome highlights the influence of the dichloroquinoline moiety on the reaction pathway. [, ]
Q8: How does the coordination chemistry of 3,7-dichloroquinoline-8-carboxylic acid differ with various metals?
A10: The carboxylate group of 3,7-dichloroquinoline-8-carboxylic acid can coordinate with different metal ions. For instance, with cobalt(II), it forms a linear chain polymer where cobalt ions are bridged by carboxylate groups. [] In contrast, with zinc(II), it forms a mononuclear complex with a distorted square-pyramidal geometry around the zinc ion. [] These variations demonstrate the versatility of the ligand in coordinating with different metal centers, leading to diverse structural motifs.
Q9: What is the crystal structure of ethyl 3,7-dichloroquinoline-8-carboxylate?
A11: The crystal structure of ethyl 3,7-dichloroquinoline-8-carboxylate is stabilized by π–π stacking interactions between the benzene and pyridine rings of adjacent molecules. Additionally, weak intermolecular C—H⋯N hydrogen bonds contribute to the overall stability of the crystal lattice. []
Q10: Can you elaborate on the synthesis of 3,7-dichloroquinoline-8-carboxylate derivatives containing a 3-methyl-1H-pyrazol-5-yl group and their herbicidal activity?
A12: Researchers synthesized a series of 3,7-dichloroquinoline-8-carboxylate derivatives containing a 3-methyl-1H-pyrazol-5-yl group, aiming to enhance the herbicidal potency of quinclorac. They observed that the activity of these compounds was influenced by the electronic nature of the substituents on the phenyl ring, with electron-withdrawing groups generally leading to higher activity against barnyard grass. []
Q11: What is the crystal structure of a specific derivative, 1,3-dimethyl-1H-pyrazol-5-yl 3,7-dichloroquinoline-8-carboxylate (10a)?
A13: The structure of 1,3-dimethyl-1H-pyrazol-5-yl 3,7-dichloroquinoline-8-carboxylate (10a), a promising herbicide candidate, was confirmed through X-ray diffraction analysis. [] While the specific details of the crystal structure were not elaborated upon in the provided abstracts, X-ray diffraction data is crucial for understanding the molecular conformation, packing, and potential intermolecular interactions of this compound.
試験管内研究製品の免責事項と情報
BenchChemで提示されるすべての記事および製品情報は、情報提供を目的としています。BenchChemで購入可能な製品は、生体外研究のために特別に設計されています。生体外研究は、ラテン語の "in glass" に由来し、生物体の外で行われる実験を指します。これらの製品は医薬品または薬として分類されておらず、FDAから任何の医療状態、病気、または疾患の予防、治療、または治癒のために承認されていません。これらの製品を人間または動物に体内に導入する形態は、法律により厳格に禁止されています。これらのガイドラインに従うことは、研究と実験において法的および倫理的な基準の遵守を確実にするために重要です。