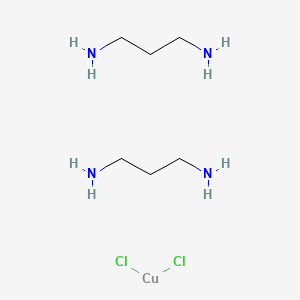
Bis(1,3-propanediamine) Copper(II) Dichloride
概要
説明
Bis(1,3-propanediamine) Copper(II) Dichloride: is a coordination compound with the chemical formula C6H20Cl2CuN4 It is known for its distinctive blue to purple color and is used in various scientific and industrial applications
準備方法
Synthetic Routes and Reaction Conditions: The preparation of Bis(1,3-propanediamine) Copper(II) Dichloride typically involves the reaction of copper(II) chloride with 1,3-propanediamine. The process can be summarized as follows:
- Dissolve copper(II) chloride in an appropriate amount of water.
- Gradually add 1,3-propanediamine to the solution while stirring.
- Adjust the pH of the solution to an appropriate level through titration.
- Filter or crystallize the resulting product to obtain this compound as a solid .
Industrial Production Methods: Industrial production of this compound follows similar steps but on a larger scale, ensuring purity and consistency. The reaction conditions are carefully controlled to optimize yield and minimize impurities.
化学反応の分析
Types of Reactions: Bis(1,3-propanediamine) Copper(II) Dichloride undergoes various chemical reactions, including:
Oxidation: It can act as a catalyst in the oxidation of hydroquinones with oxygen.
Reduction: The compound can participate in reduction reactions, often facilitated by its copper(II) center.
Substitution: Ligand substitution reactions are common, where the 1,3-propanediamine ligands can be replaced by other ligands under suitable conditions.
Common Reagents and Conditions:
Oxidation: Oxygen is a common reagent used in the presence of this compound.
Reduction: Reducing agents such as hydrazine or sodium borohydride can be used.
Substitution: Various ligands, including amines and phosphines, can be introduced to replace the existing ligands.
Major Products: The major products formed depend on the specific reaction conditions and reagents used. For example, in oxidation reactions, quinones are typically formed from hydroquinones .
科学的研究の応用
Bis(1,3-propanediamine) Copper(II) Dichloride has a wide range of applications in scientific research:
Chemistry: It is used as a catalyst in organic synthesis, particularly in oxidation reactions.
Biology: The compound’s potential in biological systems is being explored, especially in enzyme mimetics and bioinorganic chemistry.
Medicine: Research is ongoing into its potential therapeutic applications, including its role in antimicrobial and anticancer activities.
Industry: It is used in electrochemical applications, such as in the development of sensors and batteries.
作用機序
The mechanism of action of Bis(1,3-propanediamine) Copper(II) Dichloride involves its ability to coordinate with various substrates through its copper(II) center. This coordination facilitates electron transfer processes, making it an effective catalyst in oxidation and reduction reactions. The molecular targets and pathways involved include interactions with organic molecules and potential biological targets, leading to various chemical transformations .
類似化合物との比較
- Bis(ethylenediamine) Copper(II) Dichloride
- Bis(2,2’-bipyridine) Copper(II) Dichloride
- Bis(1,10-phenanthroline) Copper(II) Dichloride
Comparison: Bis(1,3-propanediamine) Copper(II) Dichloride is unique due to its specific ligand structure, which provides distinct steric and electronic properties compared to other copper(II) complexes. This uniqueness makes it particularly effective in certain catalytic applications and electrochemical processes .
特性
IUPAC Name |
dichlorocopper;propane-1,3-diamine | |
---|---|---|
Source | PubChem | |
URL | https://pubchem.ncbi.nlm.nih.gov | |
Description | Data deposited in or computed by PubChem | |
InChI |
InChI=1S/2C3H10N2.2ClH.Cu/c2*4-2-1-3-5;;;/h2*1-5H2;2*1H;/q;;;;+2/p-2 | |
Source | PubChem | |
URL | https://pubchem.ncbi.nlm.nih.gov | |
Description | Data deposited in or computed by PubChem | |
InChI Key |
HSUSKWQWIPIFEB-UHFFFAOYSA-L | |
Source | PubChem | |
URL | https://pubchem.ncbi.nlm.nih.gov | |
Description | Data deposited in or computed by PubChem | |
Canonical SMILES |
C(CN)CN.C(CN)CN.Cl[Cu]Cl | |
Source | PubChem | |
URL | https://pubchem.ncbi.nlm.nih.gov | |
Description | Data deposited in or computed by PubChem | |
Molecular Formula |
C6H20Cl2CuN4 | |
Source | PubChem | |
URL | https://pubchem.ncbi.nlm.nih.gov | |
Description | Data deposited in or computed by PubChem | |
DSSTOX Substance ID |
DTXSID50514304 | |
Record name | Propane-1,3-diamine--dichlorocopper (2/1) | |
Source | EPA DSSTox | |
URL | https://comptox.epa.gov/dashboard/DTXSID50514304 | |
Description | DSSTox provides a high quality public chemistry resource for supporting improved predictive toxicology. | |
Molecular Weight |
282.70 g/mol | |
Source | PubChem | |
URL | https://pubchem.ncbi.nlm.nih.gov | |
Description | Data deposited in or computed by PubChem | |
CAS No. |
32270-93-2 | |
Record name | Propane-1,3-diamine--dichlorocopper (2/1) | |
Source | EPA DSSTox | |
URL | https://comptox.epa.gov/dashboard/DTXSID50514304 | |
Description | DSSTox provides a high quality public chemistry resource for supporting improved predictive toxicology. | |
Record name | Bis(1,3-propanediamine) Copper(II) Dichloride | |
Source | European Chemicals Agency (ECHA) | |
URL | https://echa.europa.eu/information-on-chemicals | |
Description | The European Chemicals Agency (ECHA) is an agency of the European Union which is the driving force among regulatory authorities in implementing the EU's groundbreaking chemicals legislation for the benefit of human health and the environment as well as for innovation and competitiveness. | |
Explanation | Use of the information, documents and data from the ECHA website is subject to the terms and conditions of this Legal Notice, and subject to other binding limitations provided for under applicable law, the information, documents and data made available on the ECHA website may be reproduced, distributed and/or used, totally or in part, for non-commercial purposes provided that ECHA is acknowledged as the source: "Source: European Chemicals Agency, http://echa.europa.eu/". Such acknowledgement must be included in each copy of the material. ECHA permits and encourages organisations and individuals to create links to the ECHA website under the following cumulative conditions: Links can only be made to webpages that provide a link to the Legal Notice page. | |
Retrosynthesis Analysis
AI-Powered Synthesis Planning: Our tool employs the Template_relevance Pistachio, Template_relevance Bkms_metabolic, Template_relevance Pistachio_ringbreaker, Template_relevance Reaxys, Template_relevance Reaxys_biocatalysis model, leveraging a vast database of chemical reactions to predict feasible synthetic routes.
One-Step Synthesis Focus: Specifically designed for one-step synthesis, it provides concise and direct routes for your target compounds, streamlining the synthesis process.
Accurate Predictions: Utilizing the extensive PISTACHIO, BKMS_METABOLIC, PISTACHIO_RINGBREAKER, REAXYS, REAXYS_BIOCATALYSIS database, our tool offers high-accuracy predictions, reflecting the latest in chemical research and data.
Strategy Settings
Precursor scoring | Relevance Heuristic |
---|---|
Min. plausibility | 0.01 |
Model | Template_relevance |
Template Set | Pistachio/Bkms_metabolic/Pistachio_ringbreaker/Reaxys/Reaxys_biocatalysis |
Top-N result to add to graph | 6 |
Feasible Synthetic Routes
試験管内研究製品の免責事項と情報
BenchChemで提示されるすべての記事および製品情報は、情報提供を目的としています。BenchChemで購入可能な製品は、生体外研究のために特別に設計されています。生体外研究は、ラテン語の "in glass" に由来し、生物体の外で行われる実験を指します。これらの製品は医薬品または薬として分類されておらず、FDAから任何の医療状態、病気、または疾患の予防、治療、または治癒のために承認されていません。これらの製品を人間または動物に体内に導入する形態は、法律により厳格に禁止されています。これらのガイドラインに従うことは、研究と実験において法的および倫理的な基準の遵守を確実にするために重要です。