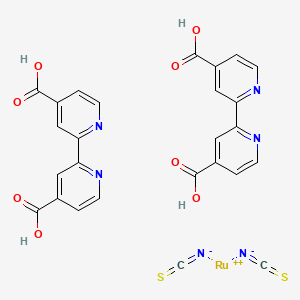
cis-Bis(isothiocyanato)bis(2,2'-bipyridyl-4,4'-dicarboxylato)ruthenium(II)
概要
説明
cis-Bis(isothiocyanato)bis(2,2’-bipyridyl-4,4’-dicarboxylato)ruthenium(II): This compound is renowned for its application in dye-sensitized solar cells (DSSCs), where it acts as a sensitizer, facilitating the conversion of sunlight into electrical energy . The compound’s structure includes two bipyridyl ligands with carboxylate groups and two isothiocyanate ligands coordinated to a central ruthenium ion, which contributes to its unique photophysical properties .
準備方法
Synthetic Routes and Reaction Conditions: The synthesis of cis-Bis(isothiocyanato)bis(2,2’-bipyridyl-4,4’-dicarboxylato)ruthenium(II) typically involves the reaction of ruthenium trichloride with 2,2’-bipyridyl-4,4’-dicarboxylic acid in the presence of a base, followed by the addition of ammonium thiocyanate . The reaction is carried out under reflux conditions, and the product is purified through recrystallization or chromatography.
Industrial Production Methods: On an industrial scale, the production of this compound involves similar synthetic routes but optimized for large-scale operations. This includes the use of continuous flow reactors to ensure consistent quality and yield. The process is designed to minimize waste and improve the efficiency of the reaction .
化学反応の分析
Types of Reactions: cis-Bis(isothiocyanato)bis(2,2’-bipyridyl-4,4’-dicarboxylato)ruthenium(II) undergoes various chemical reactions, including:
Oxidation and Reduction: The compound can participate in redox reactions, where the ruthenium center can be oxidized or reduced, altering its oxidation state.
Substitution Reactions: The isothiocyanate ligands can be substituted with other ligands under appropriate conditions.
Common Reagents and Conditions:
Oxidation: Common oxidizing agents include cerium(IV) ammonium nitrate and potassium permanganate.
Reduction: Reducing agents such as sodium borohydride and hydrazine are often used.
Substitution: Ligand exchange reactions typically involve the use of coordinating solvents or other ligands like phosphines.
Major Products: The major products of these reactions depend on the specific conditions and reagents used. For example, oxidation may yield higher oxidation state ruthenium complexes, while substitution reactions can produce a variety of ruthenium complexes with different ligands .
科学的研究の応用
Chemistry: In chemistry, cis-Bis(isothiocyanato)bis(2,2’-bipyridyl-4,4’-dicarboxylato)ruthenium(II) is extensively used in the study of photophysical and photochemical properties. It serves as a model compound for understanding electron transfer processes and the design of new photoactive materials .
Biology and Medicine: Its interaction with biological molecules is also of interest for developing new diagnostic tools .
Industry: The primary industrial application of this compound is in dye-sensitized solar cells (DSSCs). It enhances the efficiency of these cells by absorbing sunlight and converting it into electrical energy . Additionally, it is used in the development of sensors and other optoelectronic devices .
作用機序
The mechanism by which cis-Bis(isothiocyanato)bis(2,2’-bipyridyl-4,4’-dicarboxylato)ruthenium(II) exerts its effects involves the absorption of light, leading to the excitation of electrons from the ground state to an excited state . This excited state can then participate in electron transfer processes, either donating electrons to an acceptor or accepting electrons from a donor . In DSSCs, the excited electrons are injected into the conduction band of a semiconductor, typically titanium dioxide, generating an electric current .
類似化合物との比較
cis-Bis(isothiocyanato)(2,2’-bipyridyl-4,4’-dicarboxylato)(4,4’-di-nonyl-2’-bipyridyl)ruthenium(II) (Z-907): This compound is similar in structure but includes a hydrophobic bipyridyl ligand, which enhances its stability and efficiency in DSSCs.
Di-tetrabutylammonium cis-bis(isothiocyanato)bis(2,2’-bipyridyl-4,4’-dicarboxylato)ruthenium(II) (N719): This hydrophilic analogue is widely used in DSSCs for its high efficiency and stability.
Uniqueness: cis-Bis(isothiocyanato)bis(2,2’-bipyridyl-4,4’-dicarboxylato)ruthenium(II) is unique due to its balanced hydrophilic and hydrophobic properties, making it versatile for various applications. Its ability to efficiently convert sunlight into electricity while maintaining stability under different conditions sets it apart from other similar compounds .
特性
IUPAC Name |
2-(4-carboxypyridin-2-yl)pyridine-4-carboxylic acid;ruthenium(2+);dithiocyanate | |
---|---|---|
Details | Computed by LexiChem 2.6.6 (PubChem release 2019.06.18) | |
Source | PubChem | |
URL | https://pubchem.ncbi.nlm.nih.gov | |
Description | Data deposited in or computed by PubChem | |
InChI |
InChI=1S/2C12H8N2O4.2CHNS.Ru/c2*15-11(16)7-1-3-13-9(5-7)10-6-8(12(17)18)2-4-14-10;2*2-1-3;/h2*1-6H,(H,15,16)(H,17,18);2*3H;/q;;;;+2/p-2 | |
Details | Computed by InChI 1.0.5 (PubChem release 2019.06.18) | |
Source | PubChem | |
URL | https://pubchem.ncbi.nlm.nih.gov | |
Description | Data deposited in or computed by PubChem | |
InChI Key |
LTNAYKNIZNSHQA-UHFFFAOYSA-L | |
Details | Computed by InChI 1.0.5 (PubChem release 2019.06.18) | |
Source | PubChem | |
URL | https://pubchem.ncbi.nlm.nih.gov | |
Description | Data deposited in or computed by PubChem | |
Canonical SMILES |
C1=CN=C(C=C1C(=O)O)C2=NC=CC(=C2)C(=O)O.C1=CN=C(C=C1C(=O)O)C2=NC=CC(=C2)C(=O)O.C(#N)[S-].C(#N)[S-].[Ru+2] | |
Details | Computed by OEChem 2.1.5 (PubChem release 2019.06.18) | |
Source | PubChem | |
URL | https://pubchem.ncbi.nlm.nih.gov | |
Description | Data deposited in or computed by PubChem | |
Molecular Formula |
C26H16N6O8RuS2 | |
Details | Computed by PubChem 2.1 (PubChem release 2019.06.18) | |
Source | PubChem | |
URL | https://pubchem.ncbi.nlm.nih.gov | |
Description | Data deposited in or computed by PubChem | |
Molecular Weight |
705.6 g/mol | |
Details | Computed by PubChem 2.1 (PubChem release 2021.05.07) | |
Source | PubChem | |
URL | https://pubchem.ncbi.nlm.nih.gov | |
Description | Data deposited in or computed by PubChem | |
CAS No. |
141460-19-7 | |
Record name | Bis(isothiocyanato)bis(2,2'-bipyridyl-4,4'-dicarboxylato)ruthenium(II) | |
Source | European Chemicals Agency (ECHA) | |
URL | https://echa.europa.eu/information-on-chemicals | |
Description | The European Chemicals Agency (ECHA) is an agency of the European Union which is the driving force among regulatory authorities in implementing the EU's groundbreaking chemicals legislation for the benefit of human health and the environment as well as for innovation and competitiveness. | |
Explanation | Use of the information, documents and data from the ECHA website is subject to the terms and conditions of this Legal Notice, and subject to other binding limitations provided for under applicable law, the information, documents and data made available on the ECHA website may be reproduced, distributed and/or used, totally or in part, for non-commercial purposes provided that ECHA is acknowledged as the source: "Source: European Chemicals Agency, http://echa.europa.eu/". Such acknowledgement must be included in each copy of the material. ECHA permits and encourages organisations and individuals to create links to the ECHA website under the following cumulative conditions: Links can only be made to webpages that provide a link to the Legal Notice page. | |
Retrosynthesis Analysis
AI-Powered Synthesis Planning: Our tool employs the Template_relevance Pistachio, Template_relevance Bkms_metabolic, Template_relevance Pistachio_ringbreaker, Template_relevance Reaxys, Template_relevance Reaxys_biocatalysis model, leveraging a vast database of chemical reactions to predict feasible synthetic routes.
One-Step Synthesis Focus: Specifically designed for one-step synthesis, it provides concise and direct routes for your target compounds, streamlining the synthesis process.
Accurate Predictions: Utilizing the extensive PISTACHIO, BKMS_METABOLIC, PISTACHIO_RINGBREAKER, REAXYS, REAXYS_BIOCATALYSIS database, our tool offers high-accuracy predictions, reflecting the latest in chemical research and data.
Strategy Settings
Precursor scoring | Relevance Heuristic |
---|---|
Min. plausibility | 0.01 |
Model | Template_relevance |
Template Set | Pistachio/Bkms_metabolic/Pistachio_ringbreaker/Reaxys/Reaxys_biocatalysis |
Top-N result to add to graph | 6 |
Feasible Synthetic Routes
Q1: What is the molecular formula and weight of N3 dye?
A1: The molecular formula of N3 dye is C₃₂H₁₈N₈O₈RuS₂. Its molecular weight is 789.66 g/mol.
Q2: Which spectroscopic techniques are commonly used to characterize N3 dye?
A2: Common techniques include:
- UV-Vis absorption spectroscopy: Identifies characteristic absorption bands related to electronic transitions within the molecule. [, , , , ]
- FTIR spectroscopy: Provides information about the vibrational modes of the molecule, confirming the presence of specific functional groups and their interactions. [, , , , ]
- X-ray photoelectron spectroscopy (XPS): Analyzes the elemental composition and chemical states of the dye, particularly useful for studying its interaction with surfaces. [, , , , ]
- Raman spectroscopy: Investigates vibrational and rotational modes of the molecule, offering complementary information to FTIR and sensitive to changes in molecular conformation upon adsorption. [, ]
- Resonant inelastic X-ray scattering (RIXS): Provides detailed information about electronic transitions and vibrational coupling within the molecule, helpful for understanding its light-harvesting properties. [, , ]
Q3: How does N3 dye interact with titanium dioxide (TiO₂) surfaces in DSSCs?
A3: N3 dye predominantly anchors to the TiO₂ surface through the deprotonation of its carboxylic acid groups (COOH), forming strong bonds with titanium atoms. This anchoring is crucial for efficient electron injection from the dye to the TiO₂ semiconductor upon light absorption. [, , , , ]
Q4: Can the protonation state of the TiO₂ surface influence the binding of N3 dye?
A4: Yes, studies suggest that the protonation state of the TiO₂ surface can significantly impact the binding geometry of N3 dye. Protonation might induce a shift from a double bidentate-bridged binding mode to a mixed bidentate/monodentate configuration. [, ]
Q5: How does the adsorption geometry of N3 dye affect the performance of DSSCs?
A5: The efficiency of electron injection from the dye to the TiO₂ is strongly dependent on the dye's adsorption geometry. A favorable geometry promotes stronger electronic coupling, leading to faster electron transfer and higher photocurrent generation in DSSCs. [, , ]
Q6: Are there alternative substrates to TiO₂ for anchoring N3 dye in DSSCs?
A6: Yes, research has explored alternative substrates like zinc oxide (ZnO) for anchoring N3 dye. While promising, ZnO-based DSSCs often exhibit lower open-circuit voltages and efficiencies compared to TiO₂-based devices due to faster charge recombination rates. [, ]
Q7: How does the performance of N3 dye compare to other ruthenium-based dyes in DSSCs?
A7: N3 dye has historically been a benchmark for ruthenium-based sensitizers in DSSCs. While newer dyes may demonstrate higher efficiencies, N3 remains relevant due to its relatively simple synthesis and good overall performance, making it a valuable reference point for research and development. [, , ]
Q8: What are the limitations of N3 dye in DSSC applications?
A8: Some limitations of N3 dye include:
- Limited absorption in the red and near-infrared regions of the solar spectrum, which limits its light-harvesting capabilities. [, ]
- Susceptibility to degradation under prolonged exposure to light and heat, particularly in the presence of oxygen or moisture. [, , ]
- Relatively high cost compared to some emerging organic dyes. []
Q9: How have computational chemistry techniques contributed to understanding N3 dye and its interactions?
A9: Computational studies utilizing Density Functional Theory (DFT) have been instrumental in:
- Predicting the most stable adsorption configurations of N3 dye on TiO₂ and other surfaces. [, , , ]
- Calculating the electronic structure and energy levels of the dye, providing insights into its optical and electrochemical properties. [, , , ]
- Simulating the electron injection process from the dye to the semiconductor, elucidating the factors influencing charge transfer dynamics. [, , , ]
Q10: How does the structure of N3 dye relate to its activity as a sensitizer in DSSCs?
A10: Key structural features contributing to N3's activity:
- Ruthenium(II) metal center: Acts as a chromophore, absorbing light in the visible region and facilitating electron injection. [, , ]
- Bipyridyl ligands: Influence the energy levels of the dye and contribute to its light absorption properties. [, , ]
- Carboxylic acid groups: Provide strong anchoring points to the TiO₂ surface, ensuring efficient electron transfer. [, , , ]
- Isothiocyanate ligands: Can influence the dye's energy levels and electron injection dynamics. [, ]
Q11: Can modifications to the N3 dye structure enhance its performance in DSSCs?
A11: Yes, researchers have explored various modifications to improve N3 dye performance, including:
- Introducing electron-donating or electron-withdrawing groups on the bipyridyl ligands to tune the dye's absorption spectrum and energy levels. [, ]
- Modifying the length and branching of the alkyl chains on the carboxylic acid groups to influence its solubility and aggregation behavior on the TiO₂ surface. [, ]
- Replacing the isothiocyanate ligands with other functional groups to modulate electron injection dynamics. [, ]
Q12: Does N3 dye have applications beyond DSSCs?
A12: While primarily known for its role in DSSCs, N3 dye's photochemical properties have sparked interest for other applications, including:
- Photocatalysis: N3-sensitized TiO₂ has shown potential in photocatalytic degradation of organic pollutants. [, ]
Q13: What are the environmental concerns associated with N3 dye?
A13: Potential concerns include:
- Toxicity of ruthenium and its compounds, requiring careful handling and disposal procedures. []
- Use of organic solvents in the synthesis and processing of the dye, necessitating sustainable alternatives. []
Q14: Are there efforts to develop more sustainable alternatives to N3 dye?
A14: Yes, research is ongoing to develop more sustainable dyes, including:
試験管内研究製品の免責事項と情報
BenchChemで提示されるすべての記事および製品情報は、情報提供を目的としています。BenchChemで購入可能な製品は、生体外研究のために特別に設計されています。生体外研究は、ラテン語の "in glass" に由来し、生物体の外で行われる実験を指します。これらの製品は医薬品または薬として分類されておらず、FDAから任何の医療状態、病気、または疾患の予防、治療、または治癒のために承認されていません。これらの製品を人間または動物に体内に導入する形態は、法律により厳格に禁止されています。これらのガイドラインに従うことは、研究と実験において法的および倫理的な基準の遵守を確実にするために重要です。