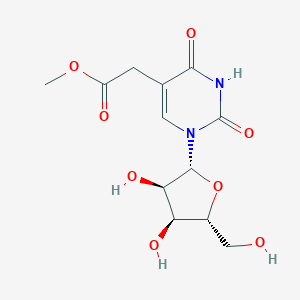
5-Methoxycarbonylmethyluridine
概要
説明
5-Methoxycarbonylmethyluridine is a derivative of uridine, a nucleoside that is a component of RNA. This compound features an additional methoxycarbonylmethyl group at the 5-position of the uracil ring. It is primarily found in the wobble position of transfer RNA (tRNA) and plays a crucial role in the accurate and efficient translation of genetic information .
科学的研究の応用
5-Methoxycarbonylmethyluridine has several scientific research applications, including:
Chemistry: It is used as a model compound for studying nucleoside modifications and their effects on RNA structure and function.
Biology: The compound is crucial for understanding the role of modified nucleosides in tRNA and their impact on protein translation.
Medicine: Research on this compound contributes to the development of therapeutic strategies targeting RNA modifications.
作用機序
5-Methoxycarbonylmethyluridine and its derivatives are found at the anticodon wobble position in several tRNAs from Gram-negative bacteria. They facilitate non-Watson–Crick base pairing with guanosine and pyrimidines at the third positions of codons, thereby expanding decoding capabilities . In humans and mammals, ALKBH8 is responsible for the final step in this compound modification in tRNAs .
将来の方向性
準備方法
Synthetic Routes and Reaction Conditions: Two novel methods have been developed for the preparation of 5-methoxycarbonylmethyluridine. The first method involves the α-hydroxylation of a 5-malonate ester derivative of uridine using selenium dioxide, followed by transformation into the corresponding methyl esters. The second approach starts from a 5-formyluridine derivative, which undergoes hydrolysis of the imidate salt prepared in an acid-catalyzed reaction with methyl alcohol .
Industrial Production Methods: While specific industrial production methods for this compound are not extensively documented, the synthetic routes mentioned above can be adapted for large-scale production with appropriate optimization of reaction conditions and purification techniques.
化学反応の分析
Types of Reactions: 5-Methoxycarbonylmethyluridine undergoes various chemical reactions, including:
Oxidation: The compound can be hydroxylated to form 5-methoxycarbonylhydroxymethyluridine.
Substitution: It can participate in substitution reactions, particularly at the 5-position of the uracil ring.
Common Reagents and Conditions:
Oxidation: Selenium dioxide is commonly used for the hydroxylation of the 5-malonate ester derivative of uridine.
Substitution: Stable Wittig reagents are employed for the formation of 5-alkyluracil derivatives.
Major Products:
類似化合物との比較
5-Carbamoylmethyluridine: Another modified uridine found in tRNA, which also plays a role in accurate protein translation.
5-Methoxycarbonylhydroxymethyluridine: A hydroxylated derivative of 5-methoxycarbonylmethyluridine, formed through oxidation reactions
Uniqueness: this compound is unique due to its specific role in the wobble position of tRNA and its involvement in the accurate decoding of genetic information. Its formation and function are tightly regulated by specific enzymes, making it a critical component of the translational machinery .
特性
IUPAC Name |
methyl 2-[1-[(2R,3R,4S,5R)-3,4-dihydroxy-5-(hydroxymethyl)oxolan-2-yl]-2,4-dioxopyrimidin-5-yl]acetate | |
---|---|---|
Source | PubChem | |
URL | https://pubchem.ncbi.nlm.nih.gov | |
Description | Data deposited in or computed by PubChem | |
InChI |
InChI=1S/C12H16N2O8/c1-21-7(16)2-5-3-14(12(20)13-10(5)19)11-9(18)8(17)6(4-15)22-11/h3,6,8-9,11,15,17-18H,2,4H2,1H3,(H,13,19,20)/t6-,8-,9-,11-/m1/s1 | |
Source | PubChem | |
URL | https://pubchem.ncbi.nlm.nih.gov | |
Description | Data deposited in or computed by PubChem | |
InChI Key |
YIZYCHKPHCPKHZ-PNHWDRBUSA-N | |
Source | PubChem | |
URL | https://pubchem.ncbi.nlm.nih.gov | |
Description | Data deposited in or computed by PubChem | |
Canonical SMILES |
COC(=O)CC1=CN(C(=O)NC1=O)C2C(C(C(O2)CO)O)O | |
Source | PubChem | |
URL | https://pubchem.ncbi.nlm.nih.gov | |
Description | Data deposited in or computed by PubChem | |
Isomeric SMILES |
COC(=O)CC1=CN(C(=O)NC1=O)[C@H]2[C@@H]([C@@H]([C@H](O2)CO)O)O | |
Source | PubChem | |
URL | https://pubchem.ncbi.nlm.nih.gov | |
Description | Data deposited in or computed by PubChem | |
Molecular Formula |
C12H16N2O8 | |
Source | PubChem | |
URL | https://pubchem.ncbi.nlm.nih.gov | |
Description | Data deposited in or computed by PubChem | |
DSSTOX Substance ID |
DTXSID20183642 | |
Record name | 5-Methoxycarbonylmethyluridine | |
Source | EPA DSSTox | |
URL | https://comptox.epa.gov/dashboard/DTXSID20183642 | |
Description | DSSTox provides a high quality public chemistry resource for supporting improved predictive toxicology. | |
Molecular Weight |
316.26 g/mol | |
Source | PubChem | |
URL | https://pubchem.ncbi.nlm.nih.gov | |
Description | Data deposited in or computed by PubChem | |
CAS No. |
29428-50-0 | |
Record name | Methyl 1,2,3,4-tetrahydro-2,4-dioxo-1-β-D-ribofuranosyl-5-pyrimidineacetate | |
Source | CAS Common Chemistry | |
URL | https://commonchemistry.cas.org/detail?cas_rn=29428-50-0 | |
Description | CAS Common Chemistry is an open community resource for accessing chemical information. Nearly 500,000 chemical substances from CAS REGISTRY cover areas of community interest, including common and frequently regulated chemicals, and those relevant to high school and undergraduate chemistry classes. This chemical information, curated by our expert scientists, is provided in alignment with our mission as a division of the American Chemical Society. | |
Explanation | The data from CAS Common Chemistry is provided under a CC-BY-NC 4.0 license, unless otherwise stated. | |
Record name | 5-Methoxycarbonylmethyluridine | |
Source | ChemIDplus | |
URL | https://pubchem.ncbi.nlm.nih.gov/substance/?source=chemidplus&sourceid=0029428500 | |
Description | ChemIDplus is a free, web search system that provides access to the structure and nomenclature authority files used for the identification of chemical substances cited in National Library of Medicine (NLM) databases, including the TOXNET system. | |
Record name | 5-Methoxycarbonylmethyluridine | |
Source | EPA DSSTox | |
URL | https://comptox.epa.gov/dashboard/DTXSID20183642 | |
Description | DSSTox provides a high quality public chemistry resource for supporting improved predictive toxicology. | |
Retrosynthesis Analysis
AI-Powered Synthesis Planning: Our tool employs the Template_relevance Pistachio, Template_relevance Bkms_metabolic, Template_relevance Pistachio_ringbreaker, Template_relevance Reaxys, Template_relevance Reaxys_biocatalysis model, leveraging a vast database of chemical reactions to predict feasible synthetic routes.
One-Step Synthesis Focus: Specifically designed for one-step synthesis, it provides concise and direct routes for your target compounds, streamlining the synthesis process.
Accurate Predictions: Utilizing the extensive PISTACHIO, BKMS_METABOLIC, PISTACHIO_RINGBREAKER, REAXYS, REAXYS_BIOCATALYSIS database, our tool offers high-accuracy predictions, reflecting the latest in chemical research and data.
Strategy Settings
Precursor scoring | Relevance Heuristic |
---|---|
Min. plausibility | 0.01 |
Model | Template_relevance |
Template Set | Pistachio/Bkms_metabolic/Pistachio_ringbreaker/Reaxys/Reaxys_biocatalysis |
Top-N result to add to graph | 6 |
Feasible Synthetic Routes
試験管内研究製品の免責事項と情報
BenchChemで提示されるすべての記事および製品情報は、情報提供を目的としています。BenchChemで購入可能な製品は、生体外研究のために特別に設計されています。生体外研究は、ラテン語の "in glass" に由来し、生物体の外で行われる実験を指します。これらの製品は医薬品または薬として分類されておらず、FDAから任何の医療状態、病気、または疾患の予防、治療、または治癒のために承認されていません。これらの製品を人間または動物に体内に導入する形態は、法律により厳格に禁止されています。これらのガイドラインに従うことは、研究と実験において法的および倫理的な基準の遵守を確実にするために重要です。