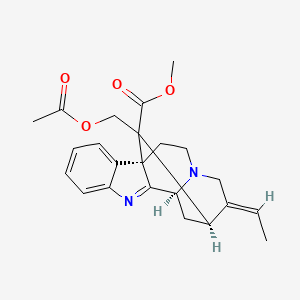
アクマミリン
概要
説明
methyl (1S,10S,12R,13E)-18-(acetyloxymethyl)-13-ethylidene-8,15-diazapentacyclo[10.5.1.01,9.02,7.010,15]octadeca-2,4,6,8-tetraene-18-carboxylate is a monoterpene indole alkaloid that belongs to a structurally diverse class of bioactive natural products. These alkaloids are primarily isolated from plants found in various parts of the world, particularly in the Apocynaceae family. The first akuammiline alkaloid, echitamine, was isolated in 1875 and first described in 1880 . methyl (1S,10S,12R,13E)-18-(acetyloxymethyl)-13-ethylidene-8,15-diazapentacyclo[10.5.1.01,9.02,7.010,15]octadeca-2,4,6,8-tetraene-18-carboxylate alkaloids are known for their complex polycyclic molecular architecture and biological activity, making them a subject of interest for organic chemists and pharmacologists .
科学的研究の応用
methyl (1S,10S,12R,13E)-18-(acetyloxymethyl)-13-ethylidene-8,15-diazapentacyclo[10.5.1.01,9.02,7.010,15]octadeca-2,4,6,8-tetraene-18-carboxylate alkaloids have a wide range of scientific research applications due to their diverse biological activities. These compounds act as ligands for various molecular targets, displaying activities such as opioid activity, cytotoxicity, and glycine receptor antagonism . They are also being investigated for their potential in treating rheumatoid arthritis by inhibiting the proliferation of fibroblast-like synoviocytes . Additionally, akuammiline alkaloids are of interest in medicinal chemistry for the development of novel physiologically active compounds .
作用機序
Akuammiline is a complex natural compound that has garnered significant attention from scientists worldwide . This article will delve into the various aspects of its mechanism of action.
Target of Action
Akuammiline alkaloids are a family of monoterpene indole alkaloids that act as ligands for a heterogeneous group of molecular targets . This broad range of targets allows them to display a wide variety of pharmacological activities .
Mode of Action
It is known that these alkaloids interact with their targets, leading to various changes in the biological system
Biochemical Pathways
The biosynthesis of Akuammiline has been decoded recently . It involves the cyclization of the common precursor geissoschizine, catalyzed by two paralogous cytochrome P450 enzymes, rhazimal synthase (AsRHS) and geissoschizine oxidase (AsGO) . These enzymes direct the metabolism towards the structurally distinct and medicinally important classes of akuammilan and strychnos alkaloids, respectively .
Result of Action
The molecular and cellular effects of Akuammiline’s action are diverse due to its interaction with a wide range of targets . For instance, some Akuammiline alkaloids have shown promising cytotoxic activity . .
生化学分析
Biochemical Properties
Akuammiline plays a crucial role in various biochemical reactions. It acts as a ligand for multiple molecular targets, displaying a wide range of pharmacological activities . Akuammiline interacts with enzymes such as cytochrome P450, which catalyzes the cyclization of geissoschizine, a precursor in the biosynthesis of akuammiline . Additionally, akuammiline has been shown to interact with opioid receptors, particularly the mu-opioid receptor, exhibiting low-affinity agonist activity . These interactions highlight the compound’s potential in modulating biochemical pathways and influencing physiological responses.
Cellular Effects
Akuammiline exerts significant effects on various cell types and cellular processes. In rheumatoid arthritis fibroblast-like synoviocytes, akuammiline derivatives have demonstrated inhibitory effects on cell proliferation . This compound influences cell function by modulating cell signaling pathways, gene expression, and cellular metabolism. For instance, akuammiline has been reported to affect the proliferation of rheumatoid arthritis fibroblast-like synoviocytes by inhibiting specific signaling pathways involved in cell growth and survival .
Molecular Mechanism
The molecular mechanism of akuammiline involves its interaction with various biomolecules. Akuammiline binds to opioid receptors, particularly the mu-opioid receptor, where it acts as a low-affinity agonist . This binding interaction leads to the modulation of downstream signaling pathways, resulting in analgesic and antimalarial effects. Additionally, akuammiline has been shown to inhibit the activity of certain enzymes, such as cytochrome P450, which plays a role in its biosynthesis . These molecular interactions contribute to the compound’s diverse pharmacological activities.
Temporal Effects in Laboratory Settings
In laboratory settings, the effects of akuammiline have been observed to change over time. Studies have shown that akuammiline exhibits stability under standard laboratory conditions, with minimal degradation over extended periods . Long-term exposure to akuammiline in in vitro and in vivo studies has revealed sustained inhibitory effects on cell proliferation and modulation of cellular functions . These findings suggest that akuammiline maintains its bioactivity over time, making it a promising candidate for further pharmacological investigations.
Dosage Effects in Animal Models
The effects of akuammiline vary with different dosages in animal models. Studies have demonstrated that akuammiline exhibits dose-dependent effects, with higher doses leading to increased pharmacological activity . At high doses, akuammiline may also exhibit toxic or adverse effects, such as hepatotoxicity and neurotoxicity . These findings highlight the importance of determining the optimal dosage range for therapeutic applications of akuammiline.
Metabolic Pathways
Akuammiline is involved in several metabolic pathways, primarily mediated by enzymes such as cytochrome P450 . The biosynthesis of akuammiline involves the cyclization of geissoschizine, catalyzed by cytochrome P450 enzymes . Additionally, akuammiline interacts with other enzymes and cofactors involved in its metabolism, influencing metabolic flux and metabolite levels . These interactions play a crucial role in determining the pharmacokinetics and pharmacodynamics of akuammiline.
Transport and Distribution
The transport and distribution of akuammiline within cells and tissues involve various transporters and binding proteins. Studies have shown that akuammiline is efficiently transported across cell membranes, facilitated by specific transporters . Once inside the cells, akuammiline is distributed to various cellular compartments, where it exerts its pharmacological effects . The localization and accumulation of akuammiline within specific tissues and organs are influenced by its interactions with binding proteins and transporters .
Subcellular Localization
Akuammiline exhibits specific subcellular localization, which influences its activity and function. Studies have shown that akuammiline is primarily localized in the cytoplasm and nucleus of cells . This subcellular distribution is mediated by targeting signals and post-translational modifications that direct akuammiline to specific compartments or organelles . The localization of akuammiline within these subcellular structures plays a crucial role in modulating its pharmacological activities and interactions with biomolecules.
準備方法
Synthetic Routes and Reaction Conditions: The synthesis of akuammiline alkaloids involves several innovative strategies and methods. One notable method is the Ag(I)-catalyzed enantioselective dearomative cyclization cascade sequence, which constructs the complex tetracyclic scaffolds from simple tryptamine and tryptophol derivatives . This method is highly efficient, yielding up to 99% and achieving enantiomeric excess greater than 99% . Another approach involves the reductive interrupted Fischer indolization reaction to construct a common pentacyclic intermediate bearing five contiguous stereocenters .
Industrial Production Methods: the development of versatile synthetic methods for constructing various skeletons of akuammiline alkaloid analogs provides a solid foundation for future industrial applications .
化学反応の分析
Types of Reactions: methyl (1S,10S,12R,13E)-18-(acetyloxymethyl)-13-ethylidene-8,15-diazapentacyclo[10.5.1.01,9.02,7.010,15]octadeca-2,4,6,8-tetraene-18-carboxylate alkaloids undergo various chemical reactions, including oxidation, reduction, and substitution. The unique indole heterocycle moiety present in these alkaloids is often involved in these reactions .
Common Reagents and Conditions: Common reagents used in the synthesis and modification of akuammiline alkaloids include electrophilic reagents for dearomatization, Ag(I) catalysts for enantioselective cyclization, and reductive agents for Fischer indolization .
Major Products Formed: The major products formed from these reactions are complex polycyclic scaffolds that serve as intermediates for further functionalization and biological evaluation .
類似化合物との比較
methyl (1S,10S,12R,13E)-18-(acetyloxymethyl)-13-ethylidene-8,15-diazapentacyclo[10.5.1.01,9.02,7.010,15]octadeca-2,4,6,8-tetraene-18-carboxylate alkaloids are compared with other monoterpene indole alkaloids, such as vallesamine and akuammicine, which also belong to the Apocynaceae family . These compounds share similar structural features but differ in their specific biological activities and molecular targets. The uniqueness of akuammiline alkaloids lies in their complex polycyclic structures and their ability to interact with a wide range of molecular targets .
List of Similar Compounds:- Vallesamine
- Akuammicine
- Bisindole alkaloids
- Quinoline alkaloids
- Isoquinoline alkaloids
Conclusion
methyl (1S,10S,12R,13E)-18-(acetyloxymethyl)-13-ethylidene-8,15-diazapentacyclo[10.5.1.01,9.02,7.010,15]octadeca-2,4,6,8-tetraene-18-carboxylate alkaloids are a fascinating class of natural products with significant potential in medicinal chemistry and pharmacology. Their complex structures and diverse biological activities make them valuable targets for synthetic and medicinal chemists. Continued research on these compounds will likely yield new insights into their mechanisms of action and potential therapeutic applications.
特性
IUPAC Name |
methyl (1S,10S,12R,13E)-18-(acetyloxymethyl)-13-ethylidene-8,15-diazapentacyclo[10.5.1.01,9.02,7.010,15]octadeca-2,4,6,8-tetraene-18-carboxylate | |
---|---|---|
Source | PubChem | |
URL | https://pubchem.ncbi.nlm.nih.gov | |
Description | Data deposited in or computed by PubChem | |
InChI |
InChI=1S/C23H26N2O4/c1-4-15-12-25-10-9-22-16-7-5-6-8-18(16)24-20(22)19(25)11-17(15)23(22,21(27)28-3)13-29-14(2)26/h4-8,17,19H,9-13H2,1-3H3/b15-4-/t17-,19+,22-,23?/m1/s1 | |
Source | PubChem | |
URL | https://pubchem.ncbi.nlm.nih.gov | |
Description | Data deposited in or computed by PubChem | |
InChI Key |
QBHALCZZZWCCLV-CLNIADLISA-N | |
Source | PubChem | |
URL | https://pubchem.ncbi.nlm.nih.gov | |
Description | Data deposited in or computed by PubChem | |
Canonical SMILES |
CC=C1CN2CCC34C5=CC=CC=C5N=C3C2CC1C4(COC(=O)C)C(=O)OC | |
Source | PubChem | |
URL | https://pubchem.ncbi.nlm.nih.gov | |
Description | Data deposited in or computed by PubChem | |
Isomeric SMILES |
C/C=C\1/CN2CC[C@@]34C5=CC=CC=C5N=C3[C@@H]2C[C@H]1C4(COC(=O)C)C(=O)OC | |
Source | PubChem | |
URL | https://pubchem.ncbi.nlm.nih.gov | |
Description | Data deposited in or computed by PubChem | |
Molecular Formula |
C23H26N2O4 | |
Source | PubChem | |
URL | https://pubchem.ncbi.nlm.nih.gov | |
Description | Data deposited in or computed by PubChem | |
Molecular Weight |
394.5 g/mol | |
Source | PubChem | |
URL | https://pubchem.ncbi.nlm.nih.gov | |
Description | Data deposited in or computed by PubChem | |
Retrosynthesis Analysis
AI-Powered Synthesis Planning: Our tool employs the Template_relevance Pistachio, Template_relevance Bkms_metabolic, Template_relevance Pistachio_ringbreaker, Template_relevance Reaxys, Template_relevance Reaxys_biocatalysis model, leveraging a vast database of chemical reactions to predict feasible synthetic routes.
One-Step Synthesis Focus: Specifically designed for one-step synthesis, it provides concise and direct routes for your target compounds, streamlining the synthesis process.
Accurate Predictions: Utilizing the extensive PISTACHIO, BKMS_METABOLIC, PISTACHIO_RINGBREAKER, REAXYS, REAXYS_BIOCATALYSIS database, our tool offers high-accuracy predictions, reflecting the latest in chemical research and data.
Strategy Settings
Precursor scoring | Relevance Heuristic |
---|---|
Min. plausibility | 0.01 |
Model | Template_relevance |
Template Set | Pistachio/Bkms_metabolic/Pistachio_ringbreaker/Reaxys/Reaxys_biocatalysis |
Top-N result to add to graph | 6 |
Feasible Synthetic Routes
Q1: What is the characteristic structural feature of akuammiline alkaloids?
A1: Akuammiline alkaloids are characterized by a methanoquinolizidine moiety, a cage-like structure. [] This structural element is related to adamantane and contributes to the unique architecture of these alkaloids.
Q2: What are some structural variations within the akuammiline alkaloid family?
A2: While the methanoquinolizidine core is consistent, akuammiline alkaloids exhibit significant structural diversity. These variations primarily stem from oxidative processes that often lead to rearrangements within the methanoquinolizidine motif. [] For example, akuammiline, strictamine, and picrinine, all share this core structure but differ in their substituents and stereochemistry.
Q3: What is the significance of the C16 stereocenter in akuammiline alkaloids?
A3: The C16 stereocenter plays a crucial role in the biological activity and biosynthesis of akuammiline alkaloids. For instance, strictamine and rhazinoline, two representative methanoquinolizidine-containing akuammiline alkaloids, differ only in their stereochemistry at the C16 position. []
Q4: What is the significance of total synthesis in understanding akuammiline alkaloids?
A4: Total synthesis of akuammiline alkaloids not only validates proposed structures but also provides access to these complex molecules and their derivatives, enabling further exploration of their biological activities and pharmacological potential. [, ]
Q5: What are some key synthetic challenges in accessing akuammiline alkaloids?
A5: The construction of the complex, polycyclic framework of akuammiline alkaloids, particularly those with a methanoquinolizidine core, presents significant synthetic challenges. The introduction of stereocenters and the formation of multiple rings require carefully orchestrated reactions and strategies. [, , ]
Q6: What are some successful synthetic approaches to akuammiline alkaloids?
A6: Several innovative strategies have been employed for the total synthesis of akuammiline alkaloids. These include:
- Interrupted Fischer Indolization: This modified version of the classic Fischer indolization reaction has proven particularly useful in constructing the fused indoline ring systems found in many akuammiline alkaloids. [, , , , , ]
- Cascade Reactions: Synthetic chemists have leveraged the intricate structures of akuammilines to develop novel cascade reactions, enabling the rapid assembly of complex molecular architectures. [, ]
- Asymmetric Catalysis: Enantioselective synthesis is crucial for accessing biologically relevant stereoisomers. Approaches utilizing chiral catalysts have enabled the synthesis of enantioenriched akuammiline alkaloids. [, , , , ]
- Biomimetic Strategies: Some syntheses draw inspiration from the proposed biosynthetic pathways of akuammilines, utilizing reactions that mimic the natural construction of these molecules. [, , ]
Q7: What is the proposed biosynthetic origin of akuammiline alkaloids?
A7: Akuammiline alkaloids are classified as monoterpenoid indole alkaloids, suggesting a biosynthetic connection to the terpenoid pathway and the amino acid tryptophan, a precursor to indole. [, ]
Q8: What role does geissoschizine play in akuammiline biosynthesis?
A8: Geissoschizine, a corynanthean alkaloid, is proposed as a key branching point in the biosynthesis of various monoterpenoid indole alkaloids, including akuammilines. "Secondary cyclizations" from geissoschizine or its derivatives are thought to lead to the diverse structures observed in this family. []
Q9: How does the double bond configuration in corynanthean alkaloids impact akuammiline biosynthesis?
A9: The E or Z configuration of the double bond at C19-C20 in corynanthean alkaloids significantly influences their ability to undergo the secondary cyclizations necessary for akuammiline formation. The E isomer, favoring a cis-quinolizidine conformation, is more prone to these cyclizations. []
Q10: What is the significance of preakuammicine in akuammiline biosynthesis?
A10: Preakuammicine has long been considered a crucial intermediate in the biosynthesis of type II and type III monoterpenoid indole alkaloids, including akuammilines, and strychnine. Its recent synthesis and characterization provide valuable insights into this complex biosynthetic pathway. []
Q11: What are some notable biological activities of akuammiline alkaloids?
A11: Akuammiline alkaloids have demonstrated a diverse array of biological activities, including:
- Cytotoxicity: Several akuammiline alkaloids exhibit potent cytotoxic effects against various cancer cell lines, suggesting potential as anticancer agents. [, ]
- Opioid Activity: Some akuammiline alkaloids, like pseudoakuammigine, display opioid activity in vivo, opening avenues for developing new analgesics. []
- Glycine Receptor Antagonism: Certain akuammiline alkaloids, such as corymine, act as antagonists of the glycine receptor, highlighting their potential in neurological disorders. []
- Multidrug Resistance Reversal: Several akuammiline alkaloids have shown promise in overcoming multidrug resistance in cancer cells, a significant challenge in chemotherapy. [, ]
Q12: What are some challenges in developing akuammiline alkaloids as therapeutics?
A12: While akuammiline alkaloids hold significant therapeutic promise, several challenges remain:
Q13: What are some promising areas for future research on akuammiline alkaloids?
A13: Future research on akuammiline alkaloids holds exciting possibilities, including:
- Structure-Activity Relationships (SAR): Systematic studies exploring how structural modifications influence biological activity are essential for optimizing pharmacological properties and designing more potent and selective analogs. []
- Drug Delivery and Targeting: Developing strategies to improve the delivery of akuammiline alkaloids to specific tissues and cells could enhance their therapeutic efficacy and reduce potential side effects. []
- Biomarkers and Diagnostics: Identifying biomarkers that predict response to akuammiline-based therapies or monitor treatment progress could revolutionize their clinical application. []
試験管内研究製品の免責事項と情報
BenchChemで提示されるすべての記事および製品情報は、情報提供を目的としています。BenchChemで購入可能な製品は、生体外研究のために特別に設計されています。生体外研究は、ラテン語の "in glass" に由来し、生物体の外で行われる実験を指します。これらの製品は医薬品または薬として分類されておらず、FDAから任何の医療状態、病気、または疾患の予防、治療、または治癒のために承認されていません。これらの製品を人間または動物に体内に導入する形態は、法律により厳格に禁止されています。これらのガイドラインに従うことは、研究と実験において法的および倫理的な基準の遵守を確実にするために重要です。