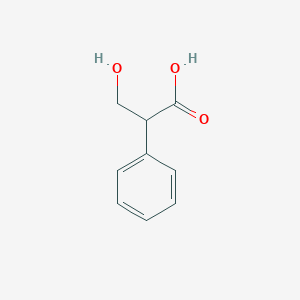
Tropic acid
概要
準備方法
合成経路および反応条件
トロピック酸は、フェニル酢酸とホルムアルデヒドのイワノフ反応によって合成できます . この方法では、グリニャール試薬であるイソプロピルマグネシウムクロリドを使用して、フェニル酢酸の二アニオンが形成され、その後、ホルムアルデヒドと反応して生成物のマグネシウム塩が形成されます。 硫酸で酸性化すると、純粋な酸が得られます .
別の方法では、アセトフェノンから出発します . トロピック酸の調製方法は、フェニル酢酸ナトリウムをマグネシウムとイソプロピルクロリドと反応させた後、得られたα-カルボキシベンジルマグネシウムクロリドのナトリウム塩をホルムアルデヒドと反応させて、得られた錯体を加水分解することを含みます .
工業的生産方法
トロピック酸の工業的生産方法は、広く文書化されていませんが、実験室設定で使用されるものと同様の合成経路が、工業的効率のために拡大されている可能性があります。
化学反応の分析
反応の種類
トロピック酸は、次のようなさまざまなタイプの化学反応を起こします。
酸化: トロピック酸は、対応するケトンまたはアルデヒドを形成するように酸化できます。
還元: 還元反応により、トロピック酸を対応するアルコールに変換できます。
置換: トロピック酸は、特にヒドロキシル基で置換反応を起こす可能性があります。
一般的な試薬と条件
酸化: 一般的な酸化剤には、過マンガン酸カリウム(KMnO₄)と三酸化クロム(CrO₃)があります。
還元: 水素化リチウムアルミニウム(LiAlH₄)や水素化ホウ素ナトリウム(NaBH₄)などの還元剤が使用されます。
置換: 置換反応では、多くの場合、ヒドロキシル基を塩化物に変換する塩化チオニル(SOCl₂)などの試薬が使用されます。
形成される主要な生成物
酸化: ケトンまたはアルデヒドの形成。
還元: アルコールの形成。
置換: 塩化物または他の置換誘導体の形成。
科学研究への応用
トロピック酸には、いくつかの科学研究への応用があります。
化学: アトロピンとヒヨスチアミンの合成における試薬として使用されます.
生物学: さまざまな生化学的経路における役割について研究されています。
医学: トロピック酸誘導体は、その抗コリン作用のために医薬品に使用されています。
産業: 特定の化学物質や医薬品の製造に使用されています。
科学的研究の応用
Tropic acid has several scientific research applications:
Chemistry: Used as a reagent in the synthesis of atropine and hyoscyamine.
Biology: Studied for its role in various biochemical pathways.
Medicine: this compound derivatives are used in pharmaceuticals for their anticholinergic properties.
Industry: Utilized in the production of certain chemicals and pharmaceuticals.
作用機序
トロピック酸は、主にアトロピンとヒヨスチアミンの合成における前駆体としての役割を通じて効果を発揮します。 これらの化合物は、ムスカリン受容体におけるアセチルコリンの競合的アンタゴニストとして作用し、副交感神経の刺激を阻害します . このメカニズムは、ムスカリン受容体に結合し、ムスカリン作動薬によって通常引き起こされるイノシトール三リン酸(IP₃)とジアシルグリセロール(DAG)の放出を阻止することを伴います .
類似の化合物との比較
類似の化合物
フェニル酢酸: 構造が似ていますが、ヒドロキシル基がありません。
ヒドロキシ酢酸: ヒドロキシル基がありますが、フェニル基がありません。
プロピオン酸: バックボーンは似ていますが、フェニル基とヒドロキシル基の両方を持っていません。
独自性
トロピック酸の独自性は、プロピオン酸バックボーンにフェニル基とヒドロキシル基の両方を含むその特定の構造にあります。 この構造により、特定の反応に参加することができ、アトロピンやヒヨスチアミンなどの重要な医薬品化合物の前駆体として役立ちます .
類似化合物との比較
Similar Compounds
Phenylacetic acid: Similar in structure but lacks the hydroxyl group.
Hydroxyacetic acid: Contains a hydroxyl group but lacks the phenyl group.
Propionic acid: Similar backbone but lacks both the phenyl and hydroxyl groups.
Uniqueness
Tropic acid’s uniqueness lies in its specific structure, which includes both a phenyl group and a hydroxyl group on the propanoic acid backbone. This structure allows it to participate in specific reactions and serve as a precursor for important pharmaceutical compounds like atropine and hyoscyamine .
生物活性
Tropic acid, a monocarboxylic acid derived from the alkaloid atropine, exhibits various biological activities that are significant in pharmacology and microbiology. This article provides a comprehensive overview of the biological activity of this compound, supported by research findings, case studies, and data tables.
This compound has the molecular formula and is characterized by its saturated structure containing an alcohol group. It can undergo transformation into athis compound upon heating, which is optically inactive . The compound is known for its role in various biochemical pathways, particularly in microbial metabolism.
Microbial Metabolism
Research indicates that certain Pseudomonas species can utilize this compound as a sole carbon source. For instance, Pseudomonas sp. strain AT3 demonstrated the ability to grow on dl-tropic acid, rapidly oxidizing it to phenylacetaldehyde and subsequently to phenylacetic acid. This metabolic pathway involves specific dehydrogenases that are NAD+-linked and exhibit optimal activity at alkaline pH levels .
Enzyme Activity
The enzymatic activity associated with this compound metabolism has been studied extensively. The following table summarizes the specific activities of key enzymes involved in this metabolic process:
Enzyme | Specific Activity (U/mg protein) | Optimal pH |
---|---|---|
This compound Dehydrogenase | 0.5 - 1.0 | 9.5 |
Phenylacetaldehyde Dehydrogenase | 0.3 - 0.7 | 7.0 |
The activity of these enzymes increases significantly upon the addition of this compound to bacterial cultures, suggesting its role as an inducer in microbial systems .
Pharmacological Implications
This compound is also relevant in pharmacology due to its structural relationship with atropine, a well-known anticholinergic agent. Its biological activity includes:
- Anticholinergic Effects : this compound contributes to the anticholinergic properties of atropine, affecting neurotransmitter pathways and potentially influencing cognitive functions .
- Antioxidant Activity : Some studies suggest that derivatives of this compound exhibit antioxidant properties, which may have implications for neuroprotection and other therapeutic applications .
Case Studies
Several studies have highlighted the significance of this compound in various biological contexts:
- Microbial Growth Study : A study involving Pseudomonas sp. strain AT3 showed that cells grown on dl-tropic acid could effectively oxidize it to phenylacetaldehyde, demonstrating the compound's role as a growth substrate .
- Antioxidant Research : Research on derivatives of this compound has indicated potential antioxidant effects, which could be beneficial in treating oxidative stress-related conditions .
- Pharmacological Analysis : Investigations into the pharmacokinetics of this compound have revealed its influence on drug metabolism and interaction with other compounds in therapeutic contexts .
特性
IUPAC Name |
3-hydroxy-2-phenylpropanoic acid | |
---|---|---|
Source | PubChem | |
URL | https://pubchem.ncbi.nlm.nih.gov | |
Description | Data deposited in or computed by PubChem | |
InChI |
InChI=1S/C9H10O3/c10-6-8(9(11)12)7-4-2-1-3-5-7/h1-5,8,10H,6H2,(H,11,12) | |
Source | PubChem | |
URL | https://pubchem.ncbi.nlm.nih.gov | |
Description | Data deposited in or computed by PubChem | |
InChI Key |
JACRWUWPXAESPB-UHFFFAOYSA-N | |
Source | PubChem | |
URL | https://pubchem.ncbi.nlm.nih.gov | |
Description | Data deposited in or computed by PubChem | |
Canonical SMILES |
C1=CC=C(C=C1)C(CO)C(=O)O | |
Source | PubChem | |
URL | https://pubchem.ncbi.nlm.nih.gov | |
Description | Data deposited in or computed by PubChem | |
Molecular Formula |
C9H10O3 | |
Source | PubChem | |
URL | https://pubchem.ncbi.nlm.nih.gov | |
Description | Data deposited in or computed by PubChem | |
DSSTOX Substance ID |
DTXSID90862179 | |
Record name | (+/-)-2-Phenyl-3-hydroxypropionic acid | |
Source | EPA DSSTox | |
URL | https://comptox.epa.gov/dashboard/DTXSID90862179 | |
Description | DSSTox provides a high quality public chemistry resource for supporting improved predictive toxicology. | |
Molecular Weight |
166.17 g/mol | |
Source | PubChem | |
URL | https://pubchem.ncbi.nlm.nih.gov | |
Description | Data deposited in or computed by PubChem | |
CAS No. |
552-63-6, 529-64-6 | |
Record name | (±)-Tropic acid | |
Source | CAS Common Chemistry | |
URL | https://commonchemistry.cas.org/detail?cas_rn=552-63-6 | |
Description | CAS Common Chemistry is an open community resource for accessing chemical information. Nearly 500,000 chemical substances from CAS REGISTRY cover areas of community interest, including common and frequently regulated chemicals, and those relevant to high school and undergraduate chemistry classes. This chemical information, curated by our expert scientists, is provided in alignment with our mission as a division of the American Chemical Society. | |
Explanation | The data from CAS Common Chemistry is provided under a CC-BY-NC 4.0 license, unless otherwise stated. | |
Record name | Tropic acid | |
Source | ChemIDplus | |
URL | https://pubchem.ncbi.nlm.nih.gov/substance/?source=chemidplus&sourceid=0000552636 | |
Description | ChemIDplus is a free, web search system that provides access to the structure and nomenclature authority files used for the identification of chemical substances cited in National Library of Medicine (NLM) databases, including the TOXNET system. | |
Record name | TROPIC ACID | |
Source | DTP/NCI | |
URL | https://dtp.cancer.gov/dtpstandard/servlet/dwindex?searchtype=NSC&outputformat=html&searchlist=20990 | |
Description | The NCI Development Therapeutics Program (DTP) provides services and resources to the academic and private-sector research communities worldwide to facilitate the discovery and development of new cancer therapeutic agents. | |
Explanation | Unless otherwise indicated, all text within NCI products is free of copyright and may be reused without our permission. Credit the National Cancer Institute as the source. | |
Record name | Benzeneacetic acid, .alpha.-(hydroxymethyl)- | |
Source | EPA Chemicals under the TSCA | |
URL | https://www.epa.gov/chemicals-under-tsca | |
Description | EPA Chemicals under the Toxic Substances Control Act (TSCA) collection contains information on chemicals and their regulations under TSCA, including non-confidential content from the TSCA Chemical Substance Inventory and Chemical Data Reporting. | |
Record name | (+/-)-2-Phenyl-3-hydroxypropionic acid | |
Source | EPA DSSTox | |
URL | https://comptox.epa.gov/dashboard/DTXSID90862179 | |
Description | DSSTox provides a high quality public chemistry resource for supporting improved predictive toxicology. | |
Record name | (±)-(hydroxymethyl)phenylacetic acid | |
Source | European Chemicals Agency (ECHA) | |
URL | https://echa.europa.eu/substance-information/-/substanceinfo/100.008.201 | |
Description | The European Chemicals Agency (ECHA) is an agency of the European Union which is the driving force among regulatory authorities in implementing the EU's groundbreaking chemicals legislation for the benefit of human health and the environment as well as for innovation and competitiveness. | |
Explanation | Use of the information, documents and data from the ECHA website is subject to the terms and conditions of this Legal Notice, and subject to other binding limitations provided for under applicable law, the information, documents and data made available on the ECHA website may be reproduced, distributed and/or used, totally or in part, for non-commercial purposes provided that ECHA is acknowledged as the source: "Source: European Chemicals Agency, http://echa.europa.eu/". Such acknowledgement must be included in each copy of the material. ECHA permits and encourages organisations and individuals to create links to the ECHA website under the following cumulative conditions: Links can only be made to webpages that provide a link to the Legal Notice page. | |
Record name | Tropic acid | |
Source | European Chemicals Agency (ECHA) | |
URL | https://echa.europa.eu/substance-information/-/substanceinfo/100.007.697 | |
Description | The European Chemicals Agency (ECHA) is an agency of the European Union which is the driving force among regulatory authorities in implementing the EU's groundbreaking chemicals legislation for the benefit of human health and the environment as well as for innovation and competitiveness. | |
Explanation | Use of the information, documents and data from the ECHA website is subject to the terms and conditions of this Legal Notice, and subject to other binding limitations provided for under applicable law, the information, documents and data made available on the ECHA website may be reproduced, distributed and/or used, totally or in part, for non-commercial purposes provided that ECHA is acknowledged as the source: "Source: European Chemicals Agency, http://echa.europa.eu/". Such acknowledgement must be included in each copy of the material. ECHA permits and encourages organisations and individuals to create links to the ECHA website under the following cumulative conditions: Links can only be made to webpages that provide a link to the Legal Notice page. | |
Record name | TROPIC ACID | |
Source | FDA Global Substance Registration System (GSRS) | |
URL | https://gsrs.ncats.nih.gov/ginas/app/beta/substances/9RM4U80765 | |
Description | The FDA Global Substance Registration System (GSRS) enables the efficient and accurate exchange of information on what substances are in regulated products. Instead of relying on names, which vary across regulatory domains, countries, and regions, the GSRS knowledge base makes it possible for substances to be defined by standardized, scientific descriptions. | |
Explanation | Unless otherwise noted, the contents of the FDA website (www.fda.gov), both text and graphics, are not copyrighted. They are in the public domain and may be republished, reprinted and otherwise used freely by anyone without the need to obtain permission from FDA. Credit to the U.S. Food and Drug Administration as the source is appreciated but not required. | |
Record name | Tropate | |
Source | Human Metabolome Database (HMDB) | |
URL | http://www.hmdb.ca/metabolites/HMDB0062590 | |
Description | The Human Metabolome Database (HMDB) is a freely available electronic database containing detailed information about small molecule metabolites found in the human body. | |
Explanation | HMDB is offered to the public as a freely available resource. Use and re-distribution of the data, in whole or in part, for commercial purposes requires explicit permission of the authors and explicit acknowledgment of the source material (HMDB) and the original publication (see the HMDB citing page). We ask that users who download significant portions of the database cite the HMDB paper in any resulting publications. | |
Retrosynthesis Analysis
AI-Powered Synthesis Planning: Our tool employs the Template_relevance Pistachio, Template_relevance Bkms_metabolic, Template_relevance Pistachio_ringbreaker, Template_relevance Reaxys, Template_relevance Reaxys_biocatalysis model, leveraging a vast database of chemical reactions to predict feasible synthetic routes.
One-Step Synthesis Focus: Specifically designed for one-step synthesis, it provides concise and direct routes for your target compounds, streamlining the synthesis process.
Accurate Predictions: Utilizing the extensive PISTACHIO, BKMS_METABOLIC, PISTACHIO_RINGBREAKER, REAXYS, REAXYS_BIOCATALYSIS database, our tool offers high-accuracy predictions, reflecting the latest in chemical research and data.
Strategy Settings
Precursor scoring | Relevance Heuristic |
---|---|
Min. plausibility | 0.01 |
Model | Template_relevance |
Template Set | Pistachio/Bkms_metabolic/Pistachio_ringbreaker/Reaxys/Reaxys_biocatalysis |
Top-N result to add to graph | 6 |
Feasible Synthetic Routes
Q1: What is the origin of tropic acid in plants?
A1: this compound biosynthesis in plants like Datura stramonium primarily originates from L-phenylalanine. Research suggests a pathway where L-phenylalanine is converted to (R)-D-phenyllactic acid, which then undergoes a rearrangement to form (S)-tropic acid. [, , , , , ]
Q2: Can you elaborate on the role of (R)-(+)-3-phenyllactic acid in this compound biosynthesis?
A2: Competitive feeding experiments in Datura species revealed that (R)-(+)-3-phenyllactic acid is a more direct precursor to this compound than phenylalanine or phenylpyruvate. [, , ] Further studies with labeled (R)-(+)-3-phenyllactic acid demonstrated its intact incorporation into the this compound moiety of hyoscyamine, confirming its role as a direct precursor. []
Q3: What is the stereochemical course of the rearrangement from (R)-(+)-3-phenyllactic acid to (S)-tropic acid?
A3: Experiments using (R,S)-DL-phenyl[2-3H]lactic acid with Datura stramonium demonstrated that the 3′-pro-R hydrogen of (R)-(+)-3-phenyllactic acid is introduced with inversion of configuration during the rearrangement to form (S)-tropic acid. This finding sheds light on the stereospecificity of the mutase enzyme involved in this crucial biosynthetic step. []
Q4: What is the chemical structure of this compound?
A4: this compound is an aromatic acid with the systematic name 3-hydroxy-2-phenylpropanoic acid.
Q5: Are there alternative biosynthetic pathways for this compound?
A5: While phenylalanine is considered the primary precursor, research suggests a minor pathway involving tryptophan. This pathway appears to be independent of the phenylalanine route. []
Q6: What is the significance of the chirality of this compound?
A6: this compound possesses a chiral center, resulting in two enantiomers: (R)-(+)-tropic acid and (S)-(−)-tropic acid. Only the (S)-(−)-enantiomer is naturally occurring and incorporated into bioactive tropane alkaloids. [, , , ]
Q7: How is atropine metabolized in different species?
A7: Atropine, a this compound ester, undergoes species-specific metabolism. In rats, phenolic metabolites like p-hydroxy-scopolamine are dominant. Rabbits primarily excrete this compound, while guinea pigs show a mixed profile with this compound and dehydrated metabolites. Mice exhibit high glucuronide conjugation of scopolamine. []
Q8: What are the key degradation products of atropine?
A8: Degradation of atropine can produce this compound, apoatropine, athis compound, and potentially other compounds depending on the conditions. The pH of the formulation significantly influences the degradation profile, with the ionized form of this compound observed at higher pH values. [, ]
Q9: What analytical techniques are used to study this compound and its derivatives?
A9: Various techniques are employed, including:
- Chromatography: Gas chromatography (GC) [, ], high-performance liquid chromatography (HPLC) [, , , ], and ultra-high performance liquid chromatography (UHPLC) [, ] are used for separation and quantification of this compound and its derivatives. Chiral chromatography is essential for separating and analyzing the enantiomers of this compound and related compounds. [, ]
- Mass Spectrometry (MS): Coupled with GC or LC, MS helps identify and characterize metabolites and degradation products based on their mass-to-charge ratios. [, , ]
- Nuclear Magnetic Resonance (NMR) Spectroscopy: Provides structural information, including stereochemistry, of this compound, its derivatives, and related compounds. [, , , , ]
- Spectrophotometry: UV spectrophotometry can be used to study the interactions between this compound derivatives and cyclodextrins, providing insight into complex formation. []
Q10: What is the toxicological profile of this compound?
A10: While generally considered non-toxic, limited research exists specifically on this compound's toxicity.
Q11: What are the potential applications of this compound?
A12: this compound's primary application lies in its use as a chiral building block for synthesizing pharmaceutical compounds, particularly anticholinergics. [, ]
Q12: Are there opportunities for utilizing this compound in material science?
A13: Research exploring the incorporation of this compound derivatives into polymers for potential biomedical applications is underway. For example, this compound cyclic esters (tropicolactones) have been used in the synthesis of sequence-controlled biodegradable copolyesters with lactic acid. []
試験管内研究製品の免責事項と情報
BenchChemで提示されるすべての記事および製品情報は、情報提供を目的としています。BenchChemで購入可能な製品は、生体外研究のために特別に設計されています。生体外研究は、ラテン語の "in glass" に由来し、生物体の外で行われる実験を指します。これらの製品は医薬品または薬として分類されておらず、FDAから任何の医療状態、病気、または疾患の予防、治療、または治癒のために承認されていません。これらの製品を人間または動物に体内に導入する形態は、法律により厳格に禁止されています。これらのガイドラインに従うことは、研究と実験において法的および倫理的な基準の遵守を確実にするために重要です。