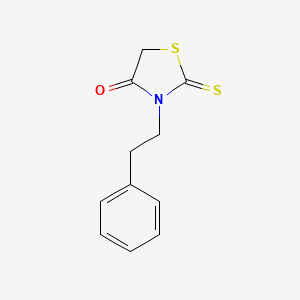
Rhodanine, 3-phenethyl-
説明
Synthesis Analysis
Rhodanine derivatives can be synthesized by the reaction of carbon disulfide, ammonia, and chloroacetic acid, which proceeds via an intermediate dithiocarbamate . Another method involves the reaction of 5- (4-hydroxy-3-methoxybenzylidene)-4-oxo-2-thioxo-1,3-thiazolidin-3-yl acetic acid and 5- (4-methoxybenzylidene)-4-oxo-2-thioxo-1,3-thiazolidin-3-yl acetic acid with different amines .Molecular Structure Analysis
The molecular structure of Rhodanine, 3-phenethyl- has been studied using X-ray structure analysis . The structure–activity relationship of rhodanine derivatives has also been discussed .Chemical Reactions Analysis
Rhodanine derivatives have been synthesized by various methods, which are applicable in the pharmaceutical and dye industries . For instance, they are known to exhibit antidiabetic activities and anti-inflammatory activities .科学的研究の応用
Anticancer Activity
3-Phenethylrhodanine has been identified as a compound with potential anticancer properties . It exhibits biological activity similar to isothiocyanates, which are known for their pronounced anticancer effects . The compound’s structure allows it to interact with various molecular targets within cancer cells, making it a candidate for further research and development in oncology.
Spectrophotometric Analysis
The compound’s quantification in pharmaceutical formulations can be achieved through spectrophotometric methods . This is crucial for ensuring the correct dosage and efficacy of the drug substance in therapeutic practice . The precision and accuracy of these methods have been validated, making them reliable for quality control.
Chromatographic Analysis (HPLC)
High-Performance Liquid Chromatography (HPLC) is another analytical technique used for the quantitative determination of 3-Phenethylrhodanine. This method is essential for the analysis of drug substances and is recommended as an equivalent alternative to spectrophotometric methods .
Structure-Activity Relationship (SAR)
Understanding the SAR of 3-Phenethylrhodanine helps in the design of new derivatives with enhanced anticancer potential. The modifications in the compound’s structure can lead to variations in its biological activity, which is pivotal for drug discovery and development .
Molecular Targets
Research into the molecular targets of 3-Phenethylrhodanine can provide insights into its mechanism of action. Identifying these targets is a key step in the development of targeted cancer therapies, which aim to minimize side effects and improve treatment outcomes .
Antibacterial Activity
Apart from its anticancer potential, 3-Phenethylrhodanine has also been explored for its antibacterial properties . This opens up possibilities for its use in treating bacterial infections, which is particularly relevant in the face of rising antibiotic resistance .
将来の方向性
Rhodanine derivatives have grabbed the attention of researchers because of their broad range of pharmacological activities. The number of scientific publications and patents describing a plenty of the different biological activities of rhodanine-based compounds is increasing continuously . This suggests that Rhodanine, 3-phenethyl- and its derivatives could be potential leads for the development of new drugs in the future .
作用機序
Target of Action
Rhodanine, 3-phenethyl-, also known as 3-Phenethylrhodanine, is a derivative of rhodanine, a five-membered heterocyclic compound . Rhodanine derivatives have been associated with numerous biological activities, including the inhibition of various targets such as kinases . These compounds have been reported to inhibit kinase’s cellular function, which can initiate and amplify cancer progression .
Mode of Action
The mode of action of 3-Phenethylrhodanine involves its interaction with its targets, primarily kinases . The compound is electrophilic and potentially reactive due to the exocyclic double bond conjugated to the carbonyl group at position 4 of the rhodanine ring . This allows for possible Michael addition of the nucleophilic protein residues to the exocyclic double bond .
Biochemical Pathways
The biochemical pathways affected by 3-Phenethylrhodanine are primarily related to kinase signaling . By inhibiting kinase activity, these compounds can alter cell signaling pathways, potentially slowing or stopping the progression of diseases like cancer .
Pharmacokinetics
It’s worth noting that the biological activity of compounds possessing a rhodanine moiety should be considered very critically despite the convincing data obtained in biological assays .
Result of Action
The result of 3-Phenethylrhodanine’s action is the inhibition of kinase activity, which can lead to alterations in cell signaling . This can potentially slow or stop the progression of diseases like cancer . .
特性
IUPAC Name |
3-(2-phenylethyl)-2-sulfanylidene-1,3-thiazolidin-4-one | |
---|---|---|
Source | PubChem | |
URL | https://pubchem.ncbi.nlm.nih.gov | |
Description | Data deposited in or computed by PubChem | |
InChI |
InChI=1S/C11H11NOS2/c13-10-8-15-11(14)12(10)7-6-9-4-2-1-3-5-9/h1-5H,6-8H2 | |
Source | PubChem | |
URL | https://pubchem.ncbi.nlm.nih.gov | |
Description | Data deposited in or computed by PubChem | |
InChI Key |
VYNFNTKJZYOOPO-UHFFFAOYSA-N | |
Source | PubChem | |
URL | https://pubchem.ncbi.nlm.nih.gov | |
Description | Data deposited in or computed by PubChem | |
Canonical SMILES |
C1C(=O)N(C(=S)S1)CCC2=CC=CC=C2 | |
Source | PubChem | |
URL | https://pubchem.ncbi.nlm.nih.gov | |
Description | Data deposited in or computed by PubChem | |
Molecular Formula |
C11H11NOS2 | |
Source | PubChem | |
URL | https://pubchem.ncbi.nlm.nih.gov | |
Description | Data deposited in or computed by PubChem | |
DSSTOX Substance ID |
DTXSID60192137 | |
Record name | Rhodanine, 3-phenethyl- | |
Source | EPA DSSTox | |
URL | https://comptox.epa.gov/dashboard/DTXSID60192137 | |
Description | DSSTox provides a high quality public chemistry resource for supporting improved predictive toxicology. | |
Molecular Weight |
237.3 g/mol | |
Source | PubChem | |
URL | https://pubchem.ncbi.nlm.nih.gov | |
Description | Data deposited in or computed by PubChem | |
CAS RN |
3889-20-1 | |
Record name | 3-Phenethyl-2-thioxothiazolidin-4-one | |
Source | CAS Common Chemistry | |
URL | https://commonchemistry.cas.org/detail?cas_rn=3889-20-1 | |
Description | CAS Common Chemistry is an open community resource for accessing chemical information. Nearly 500,000 chemical substances from CAS REGISTRY cover areas of community interest, including common and frequently regulated chemicals, and those relevant to high school and undergraduate chemistry classes. This chemical information, curated by our expert scientists, is provided in alignment with our mission as a division of the American Chemical Society. | |
Explanation | The data from CAS Common Chemistry is provided under a CC-BY-NC 4.0 license, unless otherwise stated. | |
Record name | Rhodanine, 3-phenethyl- | |
Source | ChemIDplus | |
URL | https://pubchem.ncbi.nlm.nih.gov/substance/?source=chemidplus&sourceid=0003889201 | |
Description | ChemIDplus is a free, web search system that provides access to the structure and nomenclature authority files used for the identification of chemical substances cited in National Library of Medicine (NLM) databases, including the TOXNET system. | |
Record name | Rhodanine, 3-phenethyl- | |
Source | EPA DSSTox | |
URL | https://comptox.epa.gov/dashboard/DTXSID60192137 | |
Description | DSSTox provides a high quality public chemistry resource for supporting improved predictive toxicology. | |
Q & A
Q1: What analytical methods are used to determine the purity of 3-Phenethylrhodanine?
A: Two primary analytical methods have been explored for determining the purity of 3-Phenethylrhodanine as a drug substance: spectrophotometry and high-performance liquid chromatography (HPLC) [, ]. These methods provide complementary approaches to assess the quality and purity of the compound.
Q2: Why are both spectrophotometric and chromatographic methods being compared for 3-Phenethylrhodanine analysis?
A: The comparison between spectrophotometric and HPLC methods for 3-Phenethylrhodanine analysis is crucial for several reasons [, ]. Spectrophotometry offers simplicity and cost-effectiveness, while HPLC provides higher sensitivity and separation capability for potential impurities. This comparative approach aims to identify the most suitable method for routine quality control and assurance during drug development and manufacturing.
試験管内研究製品の免責事項と情報
BenchChemで提示されるすべての記事および製品情報は、情報提供を目的としています。BenchChemで購入可能な製品は、生体外研究のために特別に設計されています。生体外研究は、ラテン語の "in glass" に由来し、生物体の外で行われる実験を指します。これらの製品は医薬品または薬として分類されておらず、FDAから任何の医療状態、病気、または疾患の予防、治療、または治癒のために承認されていません。これらの製品を人間または動物に体内に導入する形態は、法律により厳格に禁止されています。これらのガイドラインに従うことは、研究と実験において法的および倫理的な基準の遵守を確実にするために重要です。