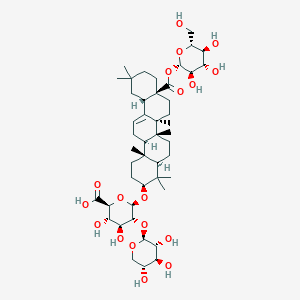
Pseudoginsenoside Rt1
説明
Pseudoginsenoside Rt1 is a triterpenoid saponin isolated from the fruit of Randia siamensis. It is known for its ichthyotoxic activity, meaning it is toxic to fish . This compound belongs to the oleanane-type saponins, which are a class of compounds with significant pharmacological activities .
科学的研究の応用
Pseudoginsenoside Rt1 has a wide range of scientific research applications:
作用機序
Target of Action
Pseudoginsenoside Rt1 is a type of ginsenoside, which are the active components of ginseng . Ginsenosides are known to interact with steroidal receptors , modulating multiple physiological activities . .
Mode of Action
Ginsenosides, including this compound, are known to exert their functions via interactions with steroidal receptors . These interactions can lead to various changes in the body, depending on the specific targets involved.
Biochemical Pathways
Ginsenosides in general are known to modulate multiple physiological activities . This suggests that this compound may affect various biochemical pathways, leading to downstream effects on the body’s physiology.
Pharmacokinetics
It’s known that the bioavailability of ginsenosides is generally low . This is one of the major hurdles that needs to be overcome to advance the use of ginsenosides, including this compound, in clinical settings .
Result of Action
This compound, isolated from the fruit of Randia siamensis, exhibits acute ichthyotoxic activity . This means it has a toxic effect on fish, which could potentially be leveraged for specific applications.
Action Environment
The action of this compound, like other ginsenosides, can be influenced by various environmental factors. For example, the quality and composition of ginsenosides in ginseng plants are influenced by factors such as the species, age, part of the plant, and cultivation conditions . .
生化学分析
Biochemical Properties
Pseudoginsenoside Rt1 plays a crucial role in various biochemical reactions. It interacts with several enzymes, proteins, and other biomolecules. For instance, it has been shown to interact with enzymes involved in cardiovascular regulation, leading to a decrease in blood pressure and an increase in heart rate . Additionally, this compound affects uterine contractility, indicating its interaction with proteins involved in muscle contraction . These interactions highlight the compound’s potential in modulating physiological processes.
Cellular Effects
This compound exerts significant effects on various cell types and cellular processes. It influences cell function by modulating cell signaling pathways, gene expression, and cellular metabolism. For example, studies have shown that this compound exhibits anti-cancer properties by inducing apoptosis in cancer cells and inhibiting cell proliferation . It also has antioxidant and anti-inflammatory effects, which contribute to its protective role in cellular health . These cellular effects underscore the compound’s potential in therapeutic applications.
Molecular Mechanism
The molecular mechanism of this compound involves several key interactions at the molecular level. It binds to specific biomolecules, leading to enzyme inhibition or activation and changes in gene expression. For instance, this compound has been shown to inhibit the activity of certain enzymes involved in inflammation, thereby reducing inflammatory responses . Additionally, it modulates gene expression by interacting with transcription factors, leading to changes in the expression of genes involved in cell survival and apoptosis . These molecular mechanisms provide insights into the compound’s therapeutic potential.
Temporal Effects in Laboratory Settings
In laboratory settings, the effects of this compound change over time. The compound’s stability and degradation play a crucial role in its long-term effects on cellular function. Studies have shown that this compound remains stable under certain conditions, allowing for sustained biological activity . Its degradation products may also exhibit biological activity, contributing to the overall effects observed in in vitro and in vivo studies . Understanding these temporal effects is essential for optimizing the compound’s therapeutic applications.
Dosage Effects in Animal Models
The effects of this compound vary with different dosages in animal models. Studies have demonstrated that low to moderate doses of the compound exhibit beneficial effects, such as improved cognitive function and reduced inflammation . High doses may lead to toxic or adverse effects, including liver damage and gastrointestinal disturbances . These dosage effects highlight the importance of determining the optimal therapeutic dose for safe and effective use.
Metabolic Pathways
This compound is involved in various metabolic pathways, interacting with enzymes and cofactors. It undergoes biotransformation in the liver, where it is metabolized by cytochrome P450 enzymes . This metabolism leads to the formation of active metabolites that contribute to the compound’s biological effects. Additionally, this compound affects metabolic flux and metabolite levels, influencing overall metabolic homeostasis . Understanding these metabolic pathways is crucial for predicting the compound’s pharmacokinetics and pharmacodynamics.
Transport and Distribution
This compound is transported and distributed within cells and tissues through specific transporters and binding proteins. It is known to interact with membrane transporters, facilitating its uptake into cells . Once inside the cells, this compound can accumulate in specific tissues, such as the liver and kidneys, where it exerts its biological effects . These transport and distribution mechanisms are essential for understanding the compound’s bioavailability and therapeutic potential.
Subcellular Localization
The subcellular localization of this compound plays a critical role in its activity and function. The compound is directed to specific cellular compartments, such as the cytoplasm and nucleus, where it interacts with target biomolecules . Post-translational modifications and targeting signals may influence its localization, affecting its biological activity . Understanding the subcellular localization of this compound provides insights into its mechanism of action and potential therapeutic applications.
準備方法
Synthetic Routes and Reaction Conditions
Pseudoginsenoside Rt1 is typically isolated from natural sources rather than synthesized chemically. The extraction process involves solvent extraction, distillation, pressing, and sublimation . The compound is then purified using chromatographic techniques.
Industrial Production Methods
Industrial production of this compound involves the extraction of total saponins from Panax japonicus, followed by biotransformation using specific glycoside hydrolases. This method allows for the efficient preparation of rare oleanane-type ginsenosides .
化学反応の分析
Types of Reactions
Pseudoginsenoside Rt1 undergoes various chemical reactions, including hydrolysis and glycosylation. The hydrolysis of the 28-O-β-d-glucopyranosyl ester bond is a common reaction for this compound .
Common Reagents and Conditions
The hydrolysis reaction typically uses glycoside hydrolases, such as PlGH3, under optimal conditions of pH 8 and 50°C . Other reagents include sodium phosphate buffer and p-nitrophenyl-β-d-glucopyranoside .
Major Products Formed
The major products formed from the hydrolysis of this compound include deglycosylated oleanane-type saponins, such as calenduloside E and zingibroside R1 .
類似化合物との比較
Pseudoginsenoside Rt1 is unique among oleanane-type saponins due to its specific glycosylation pattern. Similar compounds include:
Calenduloside E: Known for its protective effects against myocardial ischemia-reperfusion injury.
Zingibroside R1: Exhibits anti-tumor and anti-angiogenic effects.
Pseudoginsenoside Rp1: Another saponin with similar ichthyotoxic activity.
These compounds share structural similarities but differ in their specific biological activities and applications.
特性
IUPAC Name |
(2S,3S,4S,5R,6R)-6-[[(3S,4aR,6aR,6bS,8aS,12aS,14aR,14bR)-4,4,6a,6b,11,11,14b-heptamethyl-8a-[(2S,3R,4S,5S,6R)-3,4,5-trihydroxy-6-(hydroxymethyl)oxan-2-yl]oxycarbonyl-1,2,3,4a,5,6,7,8,9,10,12,12a,14,14a-tetradecahydropicen-3-yl]oxy]-3,4-dihydroxy-5-[(2S,3R,4S,5R)-3,4,5-trihydroxyoxan-2-yl]oxyoxane-2-carboxylic acid | |
---|---|---|
Source | PubChem | |
URL | https://pubchem.ncbi.nlm.nih.gov | |
Description | Data deposited in or computed by PubChem | |
InChI |
InChI=1S/C47H74O18/c1-42(2)14-16-47(41(59)65-39-34(56)30(52)29(51)24(19-48)61-39)17-15-45(6)21(22(47)18-42)8-9-26-44(5)12-11-27(43(3,4)25(44)10-13-46(26,45)7)62-40-36(32(54)31(53)35(63-40)37(57)58)64-38-33(55)28(50)23(49)20-60-38/h8,22-36,38-40,48-56H,9-20H2,1-7H3,(H,57,58)/t22-,23+,24+,25-,26+,27-,28-,29+,30-,31-,32-,33+,34+,35-,36+,38-,39-,40+,44-,45+,46+,47-/m0/s1 | |
Source | PubChem | |
URL | https://pubchem.ncbi.nlm.nih.gov | |
Description | Data deposited in or computed by PubChem | |
InChI Key |
YTPBUIWNJRGZFW-HONMPOSFSA-N | |
Source | PubChem | |
URL | https://pubchem.ncbi.nlm.nih.gov | |
Description | Data deposited in or computed by PubChem | |
Canonical SMILES |
CC1(CCC2(CCC3(C(=CCC4C3(CCC5C4(CCC(C5(C)C)OC6C(C(C(C(O6)C(=O)O)O)O)OC7C(C(C(CO7)O)O)O)C)C)C2C1)C)C(=O)OC8C(C(C(C(O8)CO)O)O)O)C | |
Source | PubChem | |
URL | https://pubchem.ncbi.nlm.nih.gov | |
Description | Data deposited in or computed by PubChem | |
Isomeric SMILES |
C[C@]12CC[C@@H](C([C@@H]1CC[C@@]3([C@@H]2CC=C4[C@]3(CC[C@@]5([C@H]4CC(CC5)(C)C)C(=O)O[C@H]6[C@@H]([C@H]([C@@H]([C@H](O6)CO)O)O)O)C)C)(C)C)O[C@H]7[C@@H]([C@H]([C@@H]([C@H](O7)C(=O)O)O)O)O[C@H]8[C@@H]([C@H]([C@@H](CO8)O)O)O | |
Source | PubChem | |
URL | https://pubchem.ncbi.nlm.nih.gov | |
Description | Data deposited in or computed by PubChem | |
Molecular Formula |
C47H74O18 | |
Source | PubChem | |
URL | https://pubchem.ncbi.nlm.nih.gov | |
Description | Data deposited in or computed by PubChem | |
Molecular Weight |
927.1 g/mol | |
Source | PubChem | |
URL | https://pubchem.ncbi.nlm.nih.gov | |
Description | Data deposited in or computed by PubChem | |
Q1: What are the sources of Pseudoginsenoside RT1?
A1: this compound has been isolated from several plant sources, including:
Q2: What is the structure of this compound?
A2: While the exact molecular formula and weight are not specified in the provided abstracts, we can infer some structural information. this compound is a saponin, meaning it has a sugar moiety attached to a non-sugar aglycone. The name "pseudoginsenoside" suggests a structural similarity to ginsenosides, which are triterpenoid saponins. Furthermore, research has identified both methyl and butyl ester derivatives of this compound [, , ], indicating the presence of a carboxyl group in the molecule. Unfortunately, the specific spectroscopic data needed for full structural elucidation is not provided in the abstracts.
Q3: What are the reported biological activities of this compound?
A3: this compound has been reported to exhibit several biological activities:
- Hypotensive activity: Studies using Randia siamensis extracts suggest that this compound contributes to the extract's hypotensive effect by potentially acting directly on blood vessels to cause vasodilation. [, , ]
- Inhibition of superoxide anion generation and elastase release: In studies on Panax japonicus var. major extracts, this compound butyl ester demonstrated potent inhibitory effects on superoxide anion generation and elastase release by human neutrophils stimulated with formyl-l-methionyl-l-leucyl-l-phenylalanine/cytochalasin B (fMLP/CB). []
Q4: How can researchers distinguish between naturally occurring this compound and potential artifacts?
A4: A study focusing on Panax japonicus var. major described the development of a LC-MS/MS method specifically designed to differentiate between naturally occurring this compound containing a butyl ester group and potentially artifactual forms of the compound. This method could be valuable for researchers working with Panax species. []
Q5: Are there any known methods for producing this compound derivatives?
A5: Yes, research has shown that Fusarium equiseti, an endophytic fungus isolated from Panax japonicus, exhibits glycoside hydrolytic activity on total saponins from the plant. This fungus can convert this compound to Pseudoginsenoside RP1 through biotransformation. This discovery presents a potential microbial approach for producing specific Pseudoginsenoside derivatives. []
試験管内研究製品の免責事項と情報
BenchChemで提示されるすべての記事および製品情報は、情報提供を目的としています。BenchChemで購入可能な製品は、生体外研究のために特別に設計されています。生体外研究は、ラテン語の "in glass" に由来し、生物体の外で行われる実験を指します。これらの製品は医薬品または薬として分類されておらず、FDAから任何の医療状態、病気、または疾患の予防、治療、または治癒のために承認されていません。これらの製品を人間または動物に体内に導入する形態は、法律により厳格に禁止されています。これらのガイドラインに従うことは、研究と実験において法的および倫理的な基準の遵守を確実にするために重要です。