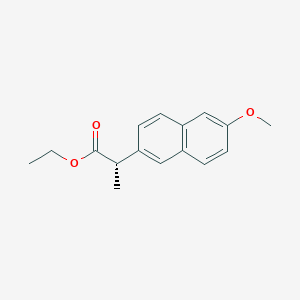
Naproxen Ethyl Ester
概要
説明
Naproxen Ethyl Ester is a derivative of naproxen, a well-known nonsteroidal anti-inflammatory drug (NSAID). This compound is synthesized by esterifying naproxen with ethanol. This compound retains the anti-inflammatory properties of naproxen but may exhibit different pharmacokinetic properties, such as improved solubility and absorption.
準備方法
Synthetic Routes and Reaction Conditions
Naproxen Ethyl Ester can be synthesized through a direct Fischer esterification reaction. This involves reacting naproxen with ethanol in the presence of an acid catalyst, such as sulfuric acid. The reaction typically occurs under reflux conditions, and the product is purified through distillation or recrystallization .
Industrial Production Methods
Industrial production of this compound follows similar principles but on a larger scale. Continuous-flow chemistry techniques can be employed to enhance the efficiency and yield of the reaction. The use of automated systems ensures consistent quality and reduces the risk of contamination .
化学反応の分析
Lipase-Catalyzed Esterification
One of the primary reactions involving naproxen ethyl ester is its synthesis through lipase-catalyzed esterification. This process utilizes enzymes, such as Candida antarctica lipase B (CALB), to facilitate the reaction in supercritical carbon dioxide, which enhances the reaction rate and conversion yields.
-
Reaction Conditions : Experiments were conducted at varying temperatures (313.15 K to 333.15 K) and pressures (50 to 175 bar) over a period of 10 hours with a stirring rate of 150 rpm.
-
Conversion Yields : The reaction demonstrated significant improvements in conversion yields when conducted under supercritical conditions compared to ambient conditions. The maximum yield achieved was approximately 68.9% in just 3 hours under optimized conditions, compared to only 9.9% over 216 hours at atmospheric pressure .
Optimization of Reaction Conditions
Statistical methods such as Response Surface Methodology (RSM) were employed to optimize the reaction parameters for maximum yield:
-
Optimum Conditions : The best results were obtained at a temperature of 325.15 K and a pressure of 130 bar, yielding approximately 67.38% conversion of this compound .
-
Effect of Enzyme and Water Content : The study also explored the impact of enzyme quantity and water content on the reaction efficiency, identifying that an optimal enzyme amount (7 g) and low water content (2%) significantly enhanced the reaction rate .
Mechanism of Lipase-Catalyzed Reactions
The mechanism involves several steps:
-
Formation of Enzyme-Substrate Complex : The lipase binds to the substrate (this compound), forming an enzyme-substrate complex.
-
Nucleophilic Attack : The hydroxyl group from the enzyme attacks the carbonyl carbon of the ester bond, facilitating the cleavage of the bond.
-
Release of Products : The final products are released, regenerating the enzyme for further catalytic cycles.
Statistical Analysis Results
Source | Sum of Squares | Degrees of Freedom | Mean Square | F-value | P>F |
---|---|---|---|---|---|
Model | 403.044 | 5 | 80.609 | 1.21 | 0.4193 |
Error | 332.802 | 5 | 66.560 | ||
Corrected Total | 735.845 | 10 | |||
Coefficient of Variation (CV) | - | - | - | - | - |
Coefficient of Determination (R²) | - | - | - | - | - |
科学的研究の応用
Synthesis and Enantioselective Resolution
Naproxen ethyl ester can be synthesized through various methods, including Fischer esterification. Recent studies have demonstrated the use of engineered esterases for the enantioselective resolution of this compound, yielding high-purity (S)-naproxen. For instance, an engineered esterase variant M3 has shown significant improvement in enantioselectivity, producing (S)-naproxen with up to 91% enantiomeric excess (ee) from racemic this compound . This biocatalytic approach is particularly valuable in the pharmaceutical industry due to its efficiency and reduced environmental impact compared to traditional chemical synthesis methods.
Analgesic and Anti-inflammatory Effects
Research indicates that this compound exhibits enhanced analgesic and anti-inflammatory activities compared to its parent compound, naproxen. In vivo studies have shown that both methyl and ethyl esters of naproxen demonstrate superior peripheral analgesia, with writhing inhibition rates of 82.09% and 82.59%, respectively, at a dose of 25 mg/kg body weight . In terms of anti-inflammatory activity, these esters achieved comparable or superior results to naproxen itself, suggesting that they may offer improved therapeutic profiles with fewer side effects.
Molecular Docking Studies
Molecular docking studies have further elucidated the mechanisms behind the enhanced activity of naproxen esters. For example, the binding energies of these compounds towards cyclooxygenase-2 (COX-2) were analyzed, revealing that certain esters exhibited better binding affinities than naproxen. This suggests a potential for developing new formulations that maximize efficacy while minimizing adverse effects .
Industrial Applications
The industrial applications of this compound are primarily centered around its use as a chiral building block in pharmaceutical synthesis. The ability to produce optically pure (S)-naproxen via biocatalysis not only streamlines the production process but also enhances the overall yield and quality of NSAIDs . Additionally, the broad substrate scope of engineered esterases makes them suitable for synthesizing various NSAIDs from their respective esters, thereby expanding their utility in drug development .
Esterase Engineering for Enhanced Selectivity
A notable case study involved the engineering of lipases to improve their enantioselectivity towards this compound. Mutations at specific amino acid sites significantly increased selectivity for the (S)-enantiomer, demonstrating a successful application of protein engineering in optimizing biocatalysts for pharmaceutical applications .
Comparative Studies on Anti-inflammatory Efficacy
In comparative studies assessing the anti-inflammatory efficacy of naproxen esters versus traditional NSAIDs, it was found that certain alkyl esters not only matched but occasionally surpassed the effectiveness of standard treatments like naproxen itself. These findings underscore the potential for developing new therapeutic agents derived from naproxen esters with improved safety profiles and reduced gastrointestinal toxicity .
作用機序
Naproxen Ethyl Ester exerts its effects by inhibiting the cyclooxygenase (COX) enzymes, similar to naproxen. This inhibition reduces the production of prostaglandins, which are mediators of inflammation and pain. The ester form may enhance the compound’s absorption and distribution in the body, potentially leading to improved therapeutic outcomes .
類似化合物との比較
Similar Compounds
- Naproxen Methyl Ester
- Naproxen Isopropyl Ester
- Ibuprofen Ethyl Ester
Comparison
Naproxen Ethyl Ester is unique due to its specific ester group, which can influence its pharmacokinetic properties. Compared to Naproxen Methyl Ester and Naproxen Isopropyl Ester, the ethyl ester may offer a balance between solubility and stability. When compared to Ibuprofen Ethyl Ester, this compound retains the anti-inflammatory properties of naproxen while potentially offering different absorption characteristics .
生物活性
Naproxen ethyl ester is a derivative of the nonsteroidal anti-inflammatory drug (NSAID) naproxen, known for its analgesic and anti-inflammatory properties. This article examines the biological activity of this compound, focusing on its pharmacological effects, synthesis, and potential applications.
Synthesis and Characterization
This compound is synthesized through the esterification of naproxen with ethanol. This reaction can be catalyzed by various methods, including enzymatic processes. For instance, studies have shown that immobilized lipases can effectively catalyze the enantioselective hydrolysis of this compound, yielding optically pure (S)-naproxen with high enantiomeric ratios (E) .
Pharmacological Activity
Analgesic and Anti-inflammatory Effects
Research indicates that this compound retains significant analgesic and anti-inflammatory activities comparable to naproxen itself. In a study involving animal models, this compound demonstrated potent peripheral analgesia with a writhing inhibition rate of 82.59%, which is higher than the 64.68% inhibition observed with naproxen at equivalent doses (25 mg/kg) .
The anti-inflammatory effects were also notable; at the fifth hour post-administration, this compound exhibited a 91.54% inhibition of inflammation compared to 95.12% for naproxen . These results suggest that the esterification does not significantly diminish the biological activity of the parent compound.
Molecular Docking Studies
Molecular docking studies have provided insights into the binding interactions of this compound with cyclooxygenase-2 (COX-2), an enzyme critical in the inflammatory pathway. The binding energy of this compound was found to be favorable, indicating strong interactions with COX-2 . The methyl and ethyl esters showed better binding energies compared to naproxen itself, suggesting enhanced efficacy in inhibiting this enzyme .
Case Studies
Enantioselective Hydrolysis
A significant case study involved the enantioselective hydrolysis of racemic this compound using immobilized lipases. The study achieved a high yield of (S)-naproxen with an enantiomeric excess exceeding 98%, demonstrating the potential for producing optically pure compounds from racemic mixtures . This process highlights the utility of biocatalysis in enhancing the pharmacological profile of NSAIDs.
Comparative Data Table
Compound | Analgesic Activity (%) | Anti-inflammatory Activity (%) | Binding Energy (kcal/mol) |
---|---|---|---|
Naproxen | 64.68 | 95.12 | -63.94 |
This compound | 82.59 | 91.54 | -65.00 (estimated) |
Naproxen Methyl Ester | 82.09 | 96.75 | -66.00 (estimated) |
特性
IUPAC Name |
ethyl (2S)-2-(6-methoxynaphthalen-2-yl)propanoate | |
---|---|---|
Source | PubChem | |
URL | https://pubchem.ncbi.nlm.nih.gov | |
Description | Data deposited in or computed by PubChem | |
InChI |
InChI=1S/C16H18O3/c1-4-19-16(17)11(2)12-5-6-14-10-15(18-3)8-7-13(14)9-12/h5-11H,4H2,1-3H3/t11-/m0/s1 | |
Source | PubChem | |
URL | https://pubchem.ncbi.nlm.nih.gov | |
Description | Data deposited in or computed by PubChem | |
InChI Key |
URNAYRDBUUZOIU-NSHDSACASA-N | |
Source | PubChem | |
URL | https://pubchem.ncbi.nlm.nih.gov | |
Description | Data deposited in or computed by PubChem | |
Canonical SMILES |
CCOC(=O)C(C)C1=CC2=C(C=C1)C=C(C=C2)OC | |
Source | PubChem | |
URL | https://pubchem.ncbi.nlm.nih.gov | |
Description | Data deposited in or computed by PubChem | |
Isomeric SMILES |
CCOC(=O)[C@@H](C)C1=CC2=C(C=C1)C=C(C=C2)OC | |
Source | PubChem | |
URL | https://pubchem.ncbi.nlm.nih.gov | |
Description | Data deposited in or computed by PubChem | |
Molecular Formula |
C16H18O3 | |
Source | PubChem | |
URL | https://pubchem.ncbi.nlm.nih.gov | |
Description | Data deposited in or computed by PubChem | |
DSSTOX Substance ID |
DTXSID20185146 | |
Record name | Naproxen ethyl ester | |
Source | EPA DSSTox | |
URL | https://comptox.epa.gov/dashboard/DTXSID20185146 | |
Description | DSSTox provides a high quality public chemistry resource for supporting improved predictive toxicology. | |
Molecular Weight |
258.31 g/mol | |
Source | PubChem | |
URL | https://pubchem.ncbi.nlm.nih.gov | |
Description | Data deposited in or computed by PubChem | |
CAS No. |
31220-35-6 | |
Record name | Naproxen ethyl ester | |
Source | ChemIDplus | |
URL | https://pubchem.ncbi.nlm.nih.gov/substance/?source=chemidplus&sourceid=0031220356 | |
Description | ChemIDplus is a free, web search system that provides access to the structure and nomenclature authority files used for the identification of chemical substances cited in National Library of Medicine (NLM) databases, including the TOXNET system. | |
Record name | Naproxen ethyl ester | |
Source | EPA DSSTox | |
URL | https://comptox.epa.gov/dashboard/DTXSID20185146 | |
Description | DSSTox provides a high quality public chemistry resource for supporting improved predictive toxicology. | |
Record name | 31220-35-6 | |
Source | European Chemicals Agency (ECHA) | |
URL | https://echa.europa.eu/information-on-chemicals | |
Description | The European Chemicals Agency (ECHA) is an agency of the European Union which is the driving force among regulatory authorities in implementing the EU's groundbreaking chemicals legislation for the benefit of human health and the environment as well as for innovation and competitiveness. | |
Explanation | Use of the information, documents and data from the ECHA website is subject to the terms and conditions of this Legal Notice, and subject to other binding limitations provided for under applicable law, the information, documents and data made available on the ECHA website may be reproduced, distributed and/or used, totally or in part, for non-commercial purposes provided that ECHA is acknowledged as the source: "Source: European Chemicals Agency, http://echa.europa.eu/". Such acknowledgement must be included in each copy of the material. ECHA permits and encourages organisations and individuals to create links to the ECHA website under the following cumulative conditions: Links can only be made to webpages that provide a link to the Legal Notice page. | |
Record name | NAPROXEN ETHYL ESTER | |
Source | FDA Global Substance Registration System (GSRS) | |
URL | https://gsrs.ncats.nih.gov/ginas/app/beta/substances/978RJD689Y | |
Description | The FDA Global Substance Registration System (GSRS) enables the efficient and accurate exchange of information on what substances are in regulated products. Instead of relying on names, which vary across regulatory domains, countries, and regions, the GSRS knowledge base makes it possible for substances to be defined by standardized, scientific descriptions. | |
Explanation | Unless otherwise noted, the contents of the FDA website (www.fda.gov), both text and graphics, are not copyrighted. They are in the public domain and may be republished, reprinted and otherwise used freely by anyone without the need to obtain permission from FDA. Credit to the U.S. Food and Drug Administration as the source is appreciated but not required. | |
Synthesis routes and methods I
Procedure details
Synthesis routes and methods II
Procedure details
Retrosynthesis Analysis
AI-Powered Synthesis Planning: Our tool employs the Template_relevance Pistachio, Template_relevance Bkms_metabolic, Template_relevance Pistachio_ringbreaker, Template_relevance Reaxys, Template_relevance Reaxys_biocatalysis model, leveraging a vast database of chemical reactions to predict feasible synthetic routes.
One-Step Synthesis Focus: Specifically designed for one-step synthesis, it provides concise and direct routes for your target compounds, streamlining the synthesis process.
Accurate Predictions: Utilizing the extensive PISTACHIO, BKMS_METABOLIC, PISTACHIO_RINGBREAKER, REAXYS, REAXYS_BIOCATALYSIS database, our tool offers high-accuracy predictions, reflecting the latest in chemical research and data.
Strategy Settings
Precursor scoring | Relevance Heuristic |
---|---|
Min. plausibility | 0.01 |
Model | Template_relevance |
Template Set | Pistachio/Bkms_metabolic/Pistachio_ringbreaker/Reaxys/Reaxys_biocatalysis |
Top-N result to add to graph | 6 |
Feasible Synthetic Routes
Q1: Why is there significant research interest in the enantioselective hydrolysis of Naproxen ethyl ester?
A1: Naproxen, the active form of this compound, exhibits chirality, meaning it exists in two mirror-image forms (enantiomers) designated as R and S. Crucially, only the S-enantiomer of Naproxen possesses the desired therapeutic properties. [, , , ] Therefore, developing efficient methods to separate or selectively synthesize the S-enantiomer from the racemic mixture is crucial for maximizing drug efficacy and minimizing potential side effects associated with the inactive R-enantiomer. []
Q2: What are the common approaches explored for the enantioselective synthesis of S-Naproxen from its ester?
A2: Researchers are actively investigating two primary strategies:
- Enzymatic Resolution: This approach utilizes enzymes like lipases to selectively hydrolyze the ester bond of R-Naproxen ethyl ester, leaving the S-Naproxen ethyl ester largely untouched. This allows for the separation and purification of S-Naproxen. Studies have explored various lipases, including those from Bacillus strains and Candida antarctica lipase B (CALB). [, ]
- Catalytic Antibodies: This innovative method involves developing antibodies that can specifically bind to and catalyze the hydrolysis of R-Naproxen ethyl ester. Researchers have successfully generated monoclonal and polyclonal antibodies demonstrating this capability. [, , ]
Q3: How does the reaction environment influence the efficiency of enzymatic resolution of this compound?
A3: Studies highlight that the reaction environment significantly impacts enzymatic activity and selectivity. For instance, conducting the lipase-catalyzed esterification of S-Naproxen ethyl ester in supercritical carbon dioxide (scCO2) demonstrated significantly improved conversion rates and reaction times compared to ambient conditions. [] This enhancement is attributed to the unique properties of scCO2, such as enhanced mass transfer and altered solvent behavior, which can positively influence enzyme activity. []
Q4: What is the significance of using haptens in generating catalytic antibodies for this compound hydrolysis?
A4: Haptens are small molecules designed to mimic the transition state of a desired chemical reaction. In the context of this compound, researchers designed a phosphonate hapten resembling the transition state of its hydrolysis. [] By conjugating this hapten to carrier proteins and using it to immunize mice, researchers could elicit the production of antibodies with a binding site complementary to the transition state of R-Naproxen ethyl ester hydrolysis. [] This approach leverages the immune system's natural ability to generate highly specific antibodies, tailoring them for catalytic activity.
試験管内研究製品の免責事項と情報
BenchChemで提示されるすべての記事および製品情報は、情報提供を目的としています。BenchChemで購入可能な製品は、生体外研究のために特別に設計されています。生体外研究は、ラテン語の "in glass" に由来し、生物体の外で行われる実験を指します。これらの製品は医薬品または薬として分類されておらず、FDAから任何の医療状態、病気、または疾患の予防、治療、または治癒のために承認されていません。これらの製品を人間または動物に体内に導入する形態は、法律により厳格に禁止されています。これらのガイドラインに従うことは、研究と実験において法的および倫理的な基準の遵守を確実にするために重要です。