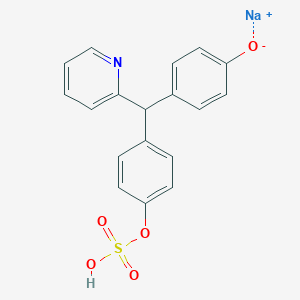
ピコモノスルファートナトリウム
- 専門家チームからの見積もりを受け取るには、QUICK INQUIRYをクリックしてください。
- 品質商品を競争力のある価格で提供し、研究に集中できます。
説明
Picomonosulfate sodium, also known as sodium picosulfate, is a stimulant laxative used primarily for the treatment of constipation and for bowel cleansing before medical procedures such as colonoscopy. It is a prodrug that is metabolized in the colon to its active form, which stimulates bowel movements by increasing peristalsis.
科学的研究の応用
Picomonosulfate sodium has a wide range of scientific research applications, including:
Chemistry: It is used as a reagent in various chemical reactions and as a model compound for studying reaction mechanisms.
Biology: It is used to study the effects of laxatives on the gastrointestinal system and to investigate the metabolism of prodrugs.
Medicine: It is used in clinical settings for bowel cleansing before procedures and for treating constipation.
Industry: It is used in the pharmaceutical industry for the production of laxative formulations
作用機序
Target of Action
Picomonosulfate sodium, also known as Sodium picosulfate, is primarily used as a stimulant laxative . Its primary targets are the mucosa of the large intestine and the rectum . These targets play a crucial role in the regulation of bowel movements and water-electrolyte balance in the body .
Mode of Action
Picomonosulfate sodium works by inhibiting the absorption of water and electrolytes , and increasing their secretion into the intestinal lumen . It is hydrolyzed by a colonic bacterial enzyme, sulfatase 2, to form an active metabolite bis-(p-hydroxy-phenyl)-pyridyl-2-methane (BHPM), which acts directly on the colonic mucosa to stimulate colonic peristalsis .
Biochemical Pathways
It is known that the compound’s action involves thestimulation of peristalsis in the gut . This is likely to involve multiple signaling pathways that regulate muscle contraction and relaxation in the intestinal wall. The downstream effects include increased bowel movements and the facilitation of stool passage.
Pharmacokinetics
It is known that the compound is aprodrug , meaning it is metabolized in the body to produce its active form, BHPM . This process occurs in the colon, mediated by colonic bacteria .
Result of Action
The primary result of Picomonosulfate sodium’s action is the induction of bowel movements , which helps in the treatment of constipation and in the preparation of the colon for procedures like colonoscopy . It achieves this by increasing peristalsis in the gut, leading to the evacuation of the bowel .
Action Environment
The action of Picomonosulfate sodium can be influenced by various environmental factors. For instance, the presence of certain gut bacteria is necessary for the conversion of the compound into its active form . Additionally, the compound’s efficacy may be affected by the patient’s hydration status, as adequate fluid intake is necessary to prevent dehydration due to increased water secretion into the intestine .
準備方法
Synthetic Routes and Reaction Conditions: The preparation of picomonosulfate sodium typically involves the use of bisacodyl as a starting material. The process includes a hydrolysis reaction followed by a sulfating reaction. The hydrolysis reaction breaks down bisacodyl, and the subsequent sulfating reaction introduces the sulfate groups necessary to form picomonosulfate sodium .
Industrial Production Methods: Industrial production of picomonosulfate sodium follows a similar synthetic route but is optimized for large-scale manufacturing. The process is designed to be simple and efficient, ensuring high purity of the final product. The production method meets the quality standards required by European and United States Pharmacopeia .
化学反応の分析
Types of Reactions: Picomonosulfate sodium undergoes several types of chemical reactions, including:
Oxidation: This reaction involves the loss of electrons and can lead to the formation of various oxidized products.
Reduction: This reaction involves the gain of electrons and can convert picomonosulfate sodium into different reduced forms.
Substitution: This reaction involves the replacement of one functional group with another, which can modify the chemical structure of picomonosulfate sodium.
Common Reagents and Conditions:
Oxidation: Common oxidizing agents include potassium permanganate and hydrogen peroxide.
Reduction: Common reducing agents include sodium borohydride and lithium aluminum hydride.
Substitution: Common reagents include halogens and nucleophiles under various conditions such as acidic or basic environments.
Major Products: The major products formed from these reactions depend on the specific reagents and conditions used. For example, oxidation can produce sulfoxides or sulfones, while reduction can yield sulfides .
類似化合物との比較
Bisacodyl: Another stimulant laxative with a similar mechanism of action.
Senna: A natural laxative that also stimulates bowel movements.
Docusate: A stool softener that works differently by increasing the water content in the stool.
Uniqueness: Picomonosulfate sodium is unique in its specific mechanism of action as a prodrug that is activated in the colon. This targeted activation reduces systemic side effects and makes it particularly effective for bowel cleansing before medical procedures .
特性
CAS番号 |
32500-19-9 |
---|---|
分子式 |
C18H14NNaO5S |
分子量 |
379.4 g/mol |
IUPAC名 |
sodium;[4-[(4-hydroxyphenyl)-pyridin-2-ylmethyl]phenyl] sulfate |
InChI |
InChI=1S/C18H15NO5S.Na/c20-15-8-4-13(5-9-15)18(17-3-1-2-12-19-17)14-6-10-16(11-7-14)24-25(21,22)23;/h1-12,18,20H,(H,21,22,23);/q;+1/p-1 |
InChIキー |
JHXGMACYSBECRB-UHFFFAOYSA-M |
SMILES |
C1=CC=NC(=C1)C(C2=CC=C(C=C2)[O-])C3=CC=C(C=C3)OS(=O)(=O)O.[Na+] |
異性体SMILES |
C1=CC=NC(=C1)C(C2=CC=C(C=C2)[O-])C3=CC=C(C=C3)OS(=O)(=O)O.[Na+] |
正規SMILES |
C1=CC=NC(=C1)C(C2=CC=C(C=C2)O)C3=CC=C(C=C3)OS(=O)(=O)[O-].[Na+] |
同義語 |
4-[(4-Hydroxyphenyl)-2-pyridinylmethyl]phenol 1-(Hydrogen Sulfate) Sodium Salt (1:1); _x000B_4,4’-(2-Pyridylmethylene)di-phenol Mono(hydrogen sulfate) Ester Monosodium Salt; 4-[(4-Hydroxyphenyl)-2-pyridinylmethyl]phenol 1-(Hydrogen sulfate) Monosodium Salt |
製品の起源 |
United States |
試験管内研究製品の免責事項と情報
BenchChemで提示されるすべての記事および製品情報は、情報提供を目的としています。BenchChemで購入可能な製品は、生体外研究のために特別に設計されています。生体外研究は、ラテン語の "in glass" に由来し、生物体の外で行われる実験を指します。これらの製品は医薬品または薬として分類されておらず、FDAから任何の医療状態、病気、または疾患の予防、治療、または治癒のために承認されていません。これらの製品を人間または動物に体内に導入する形態は、法律により厳格に禁止されています。これらのガイドラインに従うことは、研究と実験において法的および倫理的な基準の遵守を確実にするために重要です。