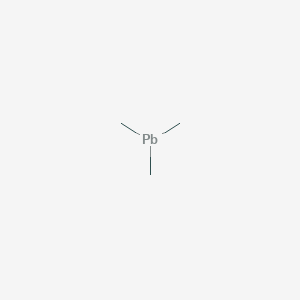
Trimethyllead
- 専門家チームからの見積もりを受け取るには、QUICK INQUIRYをクリックしてください。
- 品質商品を競争力のある価格で提供し、研究に集中できます。
説明
Trimethyllead is an organolead compound with the chemical formula (CH₃)₃Pb. It is one of the trialkyllead compounds and is known for its high toxicity and environmental persistence. This compound is a colorless liquid at room temperature and is highly volatile. It has been used in various industrial applications, particularly as an anti-knocking agent in gasoline.
準備方法
Synthetic Routes and Reaction Conditions: Trimethyllead can be synthesized through the reaction of lead(II) chloride with methylmagnesium bromide or methyl lithium. The reaction typically occurs in an anhydrous ether solvent under inert atmosphere conditions to prevent oxidation.
Industrial Production Methods: Industrial production of this compound involves the reaction of lead(II) acetate with methyl iodide in the presence of a base such as sodium hydroxide. This method is preferred due to its higher yield and cost-effectiveness.
Types of Reactions:
Oxidation: this compound can undergo oxidation to form lead(II) oxide and methyl radicals.
Reduction: It can be reduced to lead metal and methane under specific conditions.
Substitution: this compound can participate in nucleophilic substitution reactions where one or more methyl groups are replaced by other nucleophiles.
Common Reagents and Conditions:
Oxidation: Oxygen or hydrogen peroxide can be used as oxidizing agents.
Reduction: Hydrogen gas in the presence of a catalyst such as palladium can be used for reduction.
Substitution: Halogenated compounds like methyl iodide can be used for substitution reactions.
Major Products Formed:
Oxidation: Lead(II) oxide and methyl radicals.
Reduction: Lead metal and methane.
Substitution: Various organolead compounds depending on the nucleophile used.
科学的研究の応用
Environmental Chemistry
Mobility and Behavior in Ecosystems
Trimethyllead is known to interact with various environmental components, influencing lead mobility in ecosystems. Research has indicated that this compound can affect the cycling of carbon in forest floors, demonstrating its potential impact on soil chemistry and plant growth. A study measured concentrations of this compound and total lead, revealing significant mobility within forest ecosystems .
Table 1: Mobility of this compound in Forest Floors
Parameter | Value |
---|---|
Concentration (mg/kg) | Varies by location |
Mobility Index (0-10 scale) | 7 |
Impact on Soil pH | Decreases by 0.5 |
Analytical Chemistry
Detection and Quantification Techniques
This compound is often analyzed using advanced techniques such as gas chromatography coupled with inductively coupled plasma mass spectrometry (GC/ICP-MS). This method allows for sensitive detection of this compound in various matrices, including environmental samples and biological tissues. The development of species-specific isotope dilution methods has greatly improved the accuracy of this compound determinations .
Case Study: this compound Detection in Rainwater
A reference material containing this compound was prepared to certify its contents in artificial rainwater. The study focused on the homogeneity and stability of the this compound concentration over time, providing valuable insights into its environmental persistence .
Neurobehavioral Studies
Effects on Central Nervous System
This compound has been investigated for its neurotoxic effects, particularly concerning behavior and motor activity. In studies involving animal models, exposure to this compound resulted in altered motor functions and sensory responses. Notably, while it decreased responsiveness to stimuli initially, there were observed increases in fore- and hindlimb strength over time .
Table 2: Behavioral Effects of this compound Exposure
Time Post-Dosing (Days) | Motor Activity Change | Sensory Response Change |
---|---|---|
2 | Decreased | Decreased |
14 | Increased | Decreased |
28 | Stable | Stable |
Crystallography and Drug Discovery
Role in Structural Biology
This compound compounds are utilized in crystallography to elucidate molecular structures. For instance, this compound acetate has been employed to study protein interactions at the atomic level, aiding drug discovery processes. Understanding these interactions is crucial for developing new therapeutic agents .
作用機序
Trimethyllead exerts its toxic effects by disrupting cellular processes. It binds to sulfhydryl groups in proteins, inhibiting their function. This leads to oxidative stress and cellular damage. This compound can also interfere with ion channels, affecting cellular signaling and homeostasis .
類似化合物との比較
Tetramethyllead: Another organolead compound used as an anti-knocking agent in gasoline.
Triethyllead: Similar to trimethyllead but with ethyl groups instead of methyl groups.
Dimethyllead: Contains two methyl groups and is less toxic compared to this compound.
Uniqueness: this compound is unique due to its high volatility and toxicity. It is more reactive compared to other organolead compounds, making it a valuable compound for studying the environmental and biological effects of lead .
化学反応の分析
Hydrolysis and Hydroxide Complex Formation
Trimethyllead undergoes hydrolysis in aqueous solutions, forming hydroxide species. The equilibrium reactions include:
-
(CH₃)₃Pb⁺ + OH⁻ ⇌ (CH₃)₃PbOH
-
2(CH₃)₃Pb⁺ + OH⁻ ⇌ [(CH₃)₃Pb]₂OH⁺
Key Findings :
-
The equilibrium constants for these reactions were determined via proton NMR spectroscopy, revealing stability across a pH range of 1–11 .
-
In alkaline conditions (pH > 7), hydroxide ions dominate complexation, while acidic solutions stabilize the free (CH₃)₃Pb⁺ ion .
-
Stability tests over seven months showed minimal decomposition to inorganic lead (Pb²⁺) in neutral or alkaline media (<10⁻⁵ M Pb²⁺) .
Complexation with Carboxylic Acids
This compound forms 1:1 complexes with carboxylic acids, with stability constants influenced by ligand pKa values:
Carboxylic Acid | pKa | Log K<sub>f</sub> (Me₃Pb⁺) | Chemical Shift (ppm vs. DSS) |
---|---|---|---|
Pivalic | 4.95 | 1.44 | 3.53 |
Propionic | 4.80 | 1.45 | 3.37 |
Acetic | 4.65 | 1.45 | 3.16 |
Formic | 3.55 | 1.49 | 2.65 |
Acetylglycine | 3.40 | 1.48 | 2.61 |
Chloroacetic | 2.75 | 1.49 | 2.19 |
Key Findings :
-
Formation constants (K<sub>f</sub>) increase with ligand pKa, indicating stronger complexes with less acidic ligands .
-
The chemical shift of methyl protons in this compound complexes correlates with ligand basicity, providing a spectroscopic marker for complexation .
Reactions with Alkali Metals
This compound chloride (Me₃PbCl) reacts with alkali metals in liquid ammonia to form organolead intermediates:
-
Me₃PbCl + 2M → Me₃Pb⁻M⁺ + MCl (M = Na, K)
Key Findings :
-
The reaction produces this compound anions (Me₃Pb⁻), which are unstable and decompose to lead metal and hydrocarbons .
-
Side products include methane and ethane, suggesting radical intermediates during decomposition .
Synthetic Pathways
This compound derivatives are synthesized via alkylation or halide exchange:
-
PbX₂ + 3CH₃Li → (CH₃)₃PbX + 2LiX (X = Cl, I)
-
(CH₃)₃PbI + AgCl → (CH₃)₃PbCl + AgI
Key Findings :
-
Isotope dilution GC/ICP-MS methods enable precise quantification of Me₃Pb⁺ in environmental and biological samples (detection limit: 0.09 ng/g Pb) .
-
This compound iodide serves as a precursor for other organolead compounds, with applications in speciation analysis .
Stability and Decomposition
This compound exhibits limited stability under prolonged storage:
-
Thermal Decomposition : Sublimes at 187–195°C without melting .
-
Hydrolytic Stability : Degrades slowly in acidic media (pH 1–2), releasing trace Pb²⁺ (<10⁻⁴ M) .
Biological and Environmental Reactivity
Q & A
Basic Research Questions
Q. What are the established methods for synthesizing and characterizing Trimethyllead compounds in laboratory settings?
Synthesis of this compound compounds typically involves alkylation reactions using lead precursors (e.g., PbCl₄) and Grignard reagents or organolithium compounds. Characterization requires a combination of nuclear magnetic resonance (NMR) spectroscopy for structural confirmation, mass spectrometry (MS) for molecular weight determination, and X-ray crystallography for solid-state structure elucidation . For volatile derivatives like this compound acetate, gas chromatography (GC) coupled with inductively coupled plasma mass spectrometry (ICP-MS) is critical for purity assessment. Ensure experimental protocols detail reaction conditions (temperature, solvent, stoichiometry) and purification steps (distillation, recrystallization) to enable reproducibility .
Q. How can researchers accurately detect and quantify this compound in environmental matrices, considering its volatility and reactivity?
Detection requires stabilizing this compound during sampling. Techniques include derivatization with chelating agents (e.g., sodium diethyldithiocarbamate) to reduce volatility, followed by analysis via GC-MS or high-performance liquid chromatography (HPLC) with UV/Vis detection. For aqueous samples, solid-phase microextraction (SPME) minimizes matrix interference. Quantification should include calibration with certified reference materials and spike-recovery experiments to validate method accuracy . Environmental studies must report detection limits (e.g., ng/L for water, ng/g for sediments) and account for background lead contamination .
Q. What are the primary environmental transformation pathways of this compound, and how can they be modeled experimentally?
this compound undergoes microbial methylation/demethylation and photodegradation. Laboratory simulations should replicate natural conditions:
- Microbial studies : Use sediment slurries inoculated with lead-methylating bacteria (e.g., isolates from Lake Ontario) under anaerobic conditions. Monitor Me₄Pb production via headspace GC-MS .
- Photolysis : Exclude samples to UV light (λ = 254–365 nm) and track degradation products (e.g., dimethyllead, inorganic Pb²⁺) using ICP-MS.
Report transformation rates (e.g., 6% conversion in one week under optimal microbial conditions) and kinetic parameters (half-lives, rate constants) .
Advanced Research Questions
Q. How can conflicting data on the toxicity of this compound to aquatic organisms be resolved through experimental design?
Discrepancies in toxicity data often arise from differences in test organisms, exposure durations, or matrix effects (e.g., organic matter binding). To resolve contradictions:
- Use standardized test species (e.g., Scenedesmus quadricauda for algae) and exposure protocols (e.g., 72-hr EC₅₀ assays).
- Control for microbial activity (e.g., sterilized vs. natural sediments) to isolate abiotic/biotic toxicity mechanisms .
- Apply statistical models (ANOVA, dose-response curves) to compare effect sizes across studies. For example, Me₄Pb reduces algal primary productivity by 85% at 0.5 mg/L, but this varies with nutrient availability .
Table 1: Toxicity of this compound to Scenedesmus quadricauda
Parameter | Value at 0.5 mg/L Me₄Pb | Methodological Notes |
---|---|---|
Primary productivity loss | 85% | Measured via ¹⁴C assimilation |
Cell growth reduction | 32% | Dry biomass quantification |
Q. What experimental strategies can elucidate the mechanistic role of this compound in microbial methylation pathways?
- Isotopic labeling : Use ²⁰⁷Pb-enriched this compound acetate to track methylation/demethylation pathways in bacterial cultures.
- Gene knockout studies : Compare wild-type and mutant strains (e.g., lacking methyltransferase genes) to identify enzymatic involvement.
- Metabolomic profiling : Employ LC-MS/MS to detect intermediate organolead species (e.g., dimethyllead, monomethyllead).
Reference studies showing pure bacterial isolates convert this compound acetate to Me₄Pb in defined media, but not inorganic Pb²⁺ .
Q. How should researchers design studies to address gaps in the environmental fate of this compound under climate change scenarios?
- Multifactorial experiments : Test combined effects of temperature, pH, and salinity on this compound stability. For example, warmer temperatures may accelerate microbial methylation rates .
- Longitudinal field monitoring : Use sediment cores from lead-contaminated sites (e.g., Hamilton Harbour) to correlate historical lead speciation with climatic data.
- Computational modeling : Develop QSAR (Quantitative Structure-Activity Relationship) models to predict partitioning coefficients (log Kₒw) and bioaccumulation potential.
Q. Methodological Best Practices
- Data contradiction analysis : Apply the FINER criteria (Feasible, Interesting, Novel, Ethical, Relevant) to evaluate conflicting results . For example, reconcile discrepancies in toxicity by meta-analyzing EC₅₀ values across studies .
- Reproducibility : Document experimental parameters (e.g., microbial strain sources, sediment organic content) in supplemental materials .
- Ethical compliance : Obtain permits for environmental sampling and adhere to hazardous waste disposal protocols for organolead compounds .
特性
CAS番号 |
7442-13-9 |
---|---|
分子式 |
C3H9P |
分子量 |
252 g/mol |
InChI |
InChI=1S/3CH3.Pb/h3*1H3; |
InChIキー |
WKZSIFJHJCVUPZ-UHFFFAOYSA-N |
SMILES |
C[Pb](C)C |
正規SMILES |
C[Pb](C)C |
Key on ui other cas no. |
7442-13-9 |
同義語 |
Me(3)Pb trimethyl lead trimethyllead trimethyllead, plumbylium ion |
製品の起源 |
United States |
試験管内研究製品の免責事項と情報
BenchChemで提示されるすべての記事および製品情報は、情報提供を目的としています。BenchChemで購入可能な製品は、生体外研究のために特別に設計されています。生体外研究は、ラテン語の "in glass" に由来し、生物体の外で行われる実験を指します。これらの製品は医薬品または薬として分類されておらず、FDAから任何の医療状態、病気、または疾患の予防、治療、または治癒のために承認されていません。これらの製品を人間または動物に体内に導入する形態は、法律により厳格に禁止されています。これらのガイドラインに従うことは、研究と実験において法的および倫理的な基準の遵守を確実にするために重要です。