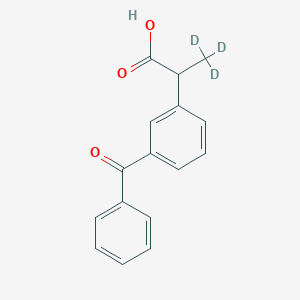
ケトプロフェン-d3
概要
説明
Ketoprofen-d3 is a deuterated form of ketoprofen, a non-steroidal anti-inflammatory drug (NSAID). It is primarily used as an analytical standard in various scientific research applications .
科学的研究の応用
Pharmacokinetics
Ketoprofen-d3 plays a critical role in pharmacokinetic studies, which investigate the absorption, distribution, metabolism, and excretion of ketoprofen in biological systems. Its deuterated nature enables researchers to differentiate between the labeled compound and its non-labeled counterpart in biological samples. This is particularly useful for:
- Studying Drug Metabolism: Understanding how ketoprofen is metabolized in various organisms.
- Bioavailability Assessments: Evaluating the extent and rate at which the active ingredient or active moiety is absorbed and becomes available at the site of action.
Analytical Chemistry
In analytical chemistry, ketoprofen-d3 serves as an internal standard in mass spectrometry and chromatography. Its application includes:
- Quantification: It provides accurate quantification of ketoprofen levels in complex biological matrices.
- Method Development: Assists in developing robust analytical methods for detecting and quantifying ketoprofen in various samples.
Biomedical Research
Ketoprofen-d3 has been extensively used in biomedical research to explore its anti-inflammatory and analgesic effects. Key applications include:
- Disease Models: Investigating the efficacy of ketoprofen in various inflammatory disease models.
- Mechanism of Action Studies: Understanding how ketoprofen inhibits cyclooxygenase (COX) enzymes, thereby reducing the synthesis of prostaglandins involved in inflammation and pain.
Environmental Studies
The compound is also relevant in environmental science for monitoring the presence and degradation of pharmaceuticals in wastewater and soil samples. Applications include:
- Environmental Monitoring: Tracking the degradation pathways of ketoprofen in different environmental matrices.
- Analytical Standardization: Used as a surrogate analytical standard for determining ketoprofen concentrations in sewage sludge samples through methods like QuEChERS (Quick, Easy, Cheap, Effective, Rugged, and Safe).
Data Table: Summary of Applications
Application Area | Specific Uses | Benefits |
---|---|---|
Pharmacokinetics | Drug metabolism studies | Differentiation between labeled and unlabeled compounds |
Analytical Chemistry | Internal standard for quantification | Improved accuracy in complex sample analysis |
Biomedical Research | Anti-inflammatory studies | Insight into mechanisms of action |
Environmental Studies | Monitoring degradation | Assessing environmental impact |
Case Studies
- Pharmacokinetic Study : A study utilized ketoprofen-d3 to evaluate its pharmacokinetics in rats. The results indicated that the deuterated form allowed for enhanced tracking of drug metabolism compared to traditional methods.
- Analytical Method Development : In a recent publication, researchers developed a new high-performance liquid chromatography (HPLC) method using ketoprofen-d3 as an internal standard to quantify ketoprofen levels in human plasma with high sensitivity and specificity.
- Environmental Impact Assessment : A study employed ketoprofen-d3 to investigate the persistence of pharmaceuticals in aquatic environments, revealing significant insights into degradation rates and potential ecological risks.
作用機序
Target of Action
Ketoprofen-d3, like its parent compound Ketoprofen, primarily targets the enzymes Prostaglandin G/H synthase 1 and 2 , also known as Cyclooxygenase-1 and -2 (COX-1 and COX-2) . These enzymes play a crucial role in the synthesis of prostaglandins, which are lipid compounds that mediate various physiological processes such as inflammation, pain, and fever .
Mode of Action
Ketoprofen-d3 exerts its therapeutic effects by inhibiting the activity of COX-1 and COX-2 enzymes . This inhibition prevents the conversion of arachidonic acid to prostaglandins, thereby reducing the inflammatory response and associated symptoms . The blocking of cyclooxygenase enzyme is reversible .
Biochemical Pathways
The primary biochemical pathway affected by Ketoprofen-d3 is the arachidonic acid pathway . By inhibiting COX-1 and COX-2, Ketoprofen-d3 disrupts the synthesis of prostaglandins from arachidonic acid . This leads to a decrease in prostaglandin levels, which in turn reduces inflammation, pain, and fever .
Result of Action
The molecular and cellular effects of Ketoprofen-d3 are primarily related to its anti-inflammatory action. By inhibiting the synthesis of prostaglandins, Ketoprofen-d3 can reduce inflammation and associated symptoms such as pain and fever . In addition, it has been shown to induce apoptosis and inhibit autophagy in certain cell lines .
Action Environment
Environmental factors can influence the action, efficacy, and stability of Ketoprofen-d3. For instance, it has been cited as an environmental contaminant that raises concerns for ecological well-being . Its presence in the environment can potentially affect various organisms and disrupt ecological functions
生化学分析
Biochemical Properties
Ketoprofen-d3, like its parent compound Ketoprofen, is known to inhibit the cyclooxygenase enzymes (COX-1 and COX-2), which are key enzymes in the synthesis of prostaglandins . Prostaglandins are bioactive lipids that play crucial roles in inflammation and pain signaling. By inhibiting these enzymes, Ketoprofen-d3 can reduce the production of prostaglandins, thereby alleviating inflammation and pain .
Cellular Effects
In cellular contexts, Ketoprofen-d3 has been shown to influence various cellular processes. For instance, it has been found to significantly reduce the ability of individual cells to produce antibodies . This suggests that Ketoprofen-d3 may have immunomodulatory effects, potentially influencing immune responses at the cellular level .
Molecular Mechanism
The molecular mechanism of action of Ketoprofen-d3 is primarily through the inhibition of the cyclooxygenase enzymes (COX-1 and COX-2) . These enzymes are involved in the conversion of arachidonic acid to prostaglandins, which are key mediators of inflammation and pain. By inhibiting these enzymes, Ketoprofen-d3 prevents the synthesis of prostaglandins, thereby exerting its anti-inflammatory and analgesic effects .
Temporal Effects in Laboratory Settings
In laboratory settings, the effects of Ketoprofen-d3 can change over time. For instance, it has been observed that the ability of an individual cell to produce antibodies and antibody-mediated immune responses is suppressed by Ketoprofen, suggesting that it is immunosuppressive .
Dosage Effects in Animal Models
In animal models, the effects of Ketoprofen-d3 can vary with different dosages. For instance, in mice, Ketoprofen at doses of 1 mg/kg/day and 5 mg/kg/day for seven days has been shown to significantly reduce the ability of individual cells to produce antibodies .
Metabolic Pathways
Ketoprofen-d3, like Ketoprofen, is involved in the arachidonic acid metabolic pathway . It interacts with the cyclooxygenase enzymes (COX-1 and COX-2), inhibiting their activity and thereby reducing the production of prostaglandins .
準備方法
Synthetic Routes and Reaction Conditions
The synthesis of Ketoprofen-d3 involves the incorporation of deuterium atoms into the ketoprofen molecule. One common method is the catalytic exchange of hydrogen atoms with deuterium in the presence of a deuterium source such as deuterium gas (D2) or deuterated solvents . The reaction typically occurs under mild conditions to ensure the selective incorporation of deuterium atoms without affecting the overall structure of the molecule .
Industrial Production Methods
Industrial production of Ketoprofen-d3 follows similar synthetic routes but on a larger scale. The process involves the use of high-pressure reactors and specialized catalysts to achieve efficient deuterium incorporation. The final product is purified using techniques such as high-performance liquid chromatography (HPLC) to ensure high purity and consistency .
化学反応の分析
Types of Reactions
Ketoprofen-d3 undergoes various chemical reactions, including:
Oxidation: The compound can be oxidized to form corresponding ketones or carboxylic acids.
Reduction: Reduction reactions can convert ketoprofen-d3 into alcohols or other reduced forms.
Substitution: Substitution reactions can replace functional groups on the benzene ring or the propionic acid moiety.
Common Reagents and Conditions
Oxidation: Common oxidizing agents include potassium permanganate (KMnO4) and chromium trioxide (CrO3).
Reduction: Reducing agents such as lithium aluminum hydride (LiAlH4) and sodium borohydride (NaBH4) are used.
Substitution: Reagents like halogens (Cl2, Br2) and nucleophiles (NH3, OH-) are employed.
Major Products Formed
Oxidation: Formation of benzophenone derivatives and carboxylic acids.
Reduction: Formation of alcohols and reduced ketones.
Substitution: Formation of halogenated or nucleophile-substituted derivatives.
類似化合物との比較
Similar Compounds
Ketoprofen: The non-deuterated form of Ketoprofen-d3, used as an NSAID for pain and inflammation.
Ibuprofen-d3: Another deuterated NSAID used as an analytical standard.
Naproxen-d3: A deuterated form of naproxen, used similarly in research.
Uniqueness
Ketoprofen-d3 is unique due to its deuterium labeling, which provides distinct advantages in analytical applications. The presence of deuterium atoms allows for more accurate quantification and tracing in mass spectrometry and other analytical techniques . This makes Ketoprofen-d3 particularly valuable in pharmacokinetic studies and environmental analysis .
生物活性
Ketoprofen-d3 is a deuterated form of ketoprofen, a non-steroidal anti-inflammatory drug (NSAID) widely used for its analgesic and anti-inflammatory properties. This article explores the biological activity of ketoprofen-d3, focusing on its pharmacokinetics, efficacy in various clinical scenarios, and its mechanism of action.
Overview of Ketoprofen-d3
Ketoprofen-d3 is characterized by the incorporation of deuterium, which can alter its pharmacokinetic properties and metabolic stability compared to its non-deuterated counterpart. Ketoprofen itself inhibits cyclooxygenase (COX) enzymes, leading to decreased synthesis of prostaglandins, which are mediators of inflammation and pain. The specific IC50 values for ketoprofen against COX-1 and COX-2 are 2 nM and 26 nM, respectively .
Pharmacokinetics
A study evaluating the pharmacokinetics of ketoprofen-d3 found significant differences in absorption and elimination rates when administered via different routes. The mean terminal elimination half-life was approximately 4.69 hours for intravenous administration compared to 25.77 hours for transdermal delivery, indicating a prolonged effect from the transdermal route .
Table 1: Pharmacokinetic Parameters of Ketoprofen-d3
Administration Route | Half-life (h) | AUC (µg·h/mL) | Bioavailability (%) |
---|---|---|---|
Intravenous | 4.69 ± 1.33 | 15.75 ± 7.72 | ~7 |
Transdermal | 25.77 ± 22.15 | 8.13 ± 4.28 | ~7 |
Efficacy in Clinical Studies
Case Study: Treatment of Clinical Mastitis in Cattle
A randomized controlled trial assessed the efficacy of ketoprofen in treating spontaneous, culture-negative clinical mastitis in dairy cows. Cows receiving an intramuscular injection of ketoprofen (3 mg/kg) showed no significant improvement in clinical cure rates or somatic cell counts compared to untreated controls . This suggests that while ketoprofen may reduce inflammation, it does not significantly impact the resolution of mastitis symptoms.
Case Study: Immune Response Modulation
Research involving lipopolysaccharide (LPS)-induced immune activation in pigs demonstrated that ketoprofen administration attenuated the behavioral signs of sickness and modulated cytokine levels . Specifically, ketoprofen reduced cortisol release and influenced neurotransmitter levels, suggesting a role in managing stress responses during inflammatory challenges.
Ketoprofen-d3 exerts its effects primarily through the inhibition of COX enzymes, leading to reduced production of pro-inflammatory prostaglandins. This mechanism is critical in alleviating pain and inflammation associated with various conditions.
Inflammatory Pathway Involvement
The modulation of inflammatory pathways by ketoprofen-d3 includes:
- Cytokine Regulation : Decreased levels of inflammatory cytokines such as TNF-α and IL-18 were observed following treatment with ketoprofen during immune challenges .
- Neurotransmitter Modulation : Ketoprofen's influence on neurotransmitter systems, particularly serotonin and dopamine, suggests potential applications in managing mood-related disorders linked to inflammation .
特性
IUPAC Name |
2-(3-benzoylphenyl)-3,3,3-trideuteriopropanoic acid | |
---|---|---|
Source | PubChem | |
URL | https://pubchem.ncbi.nlm.nih.gov | |
Description | Data deposited in or computed by PubChem | |
InChI |
InChI=1S/C16H14O3/c1-11(16(18)19)13-8-5-9-14(10-13)15(17)12-6-3-2-4-7-12/h2-11H,1H3,(H,18,19)/i1D3 | |
Source | PubChem | |
URL | https://pubchem.ncbi.nlm.nih.gov | |
Description | Data deposited in or computed by PubChem | |
InChI Key |
DKYWVDODHFEZIM-FIBGUPNXSA-N | |
Source | PubChem | |
URL | https://pubchem.ncbi.nlm.nih.gov | |
Description | Data deposited in or computed by PubChem | |
Canonical SMILES |
CC(C1=CC(=CC=C1)C(=O)C2=CC=CC=C2)C(=O)O | |
Source | PubChem | |
URL | https://pubchem.ncbi.nlm.nih.gov | |
Description | Data deposited in or computed by PubChem | |
Isomeric SMILES |
[2H]C([2H])([2H])C(C1=CC(=CC=C1)C(=O)C2=CC=CC=C2)C(=O)O | |
Source | PubChem | |
URL | https://pubchem.ncbi.nlm.nih.gov | |
Description | Data deposited in or computed by PubChem | |
Molecular Formula |
C16H14O3 | |
Source | PubChem | |
URL | https://pubchem.ncbi.nlm.nih.gov | |
Description | Data deposited in or computed by PubChem | |
Molecular Weight |
257.30 g/mol | |
Source | PubChem | |
URL | https://pubchem.ncbi.nlm.nih.gov | |
Description | Data deposited in or computed by PubChem | |
CAS No. |
159490-55-8 | |
Record name | 159490-55-8 | |
Source | European Chemicals Agency (ECHA) | |
URL | https://echa.europa.eu/information-on-chemicals | |
Description | The European Chemicals Agency (ECHA) is an agency of the European Union which is the driving force among regulatory authorities in implementing the EU's groundbreaking chemicals legislation for the benefit of human health and the environment as well as for innovation and competitiveness. | |
Explanation | Use of the information, documents and data from the ECHA website is subject to the terms and conditions of this Legal Notice, and subject to other binding limitations provided for under applicable law, the information, documents and data made available on the ECHA website may be reproduced, distributed and/or used, totally or in part, for non-commercial purposes provided that ECHA is acknowledged as the source: "Source: European Chemicals Agency, http://echa.europa.eu/". Such acknowledgement must be included in each copy of the material. ECHA permits and encourages organisations and individuals to create links to the ECHA website under the following cumulative conditions: Links can only be made to webpages that provide a link to the Legal Notice page. | |
Synthesis routes and methods I
Procedure details
Synthesis routes and methods II
Procedure details
Synthesis routes and methods III
Procedure details
Synthesis routes and methods IV
Procedure details
Synthesis routes and methods V
Procedure details
Retrosynthesis Analysis
AI-Powered Synthesis Planning: Our tool employs the Template_relevance Pistachio, Template_relevance Bkms_metabolic, Template_relevance Pistachio_ringbreaker, Template_relevance Reaxys, Template_relevance Reaxys_biocatalysis model, leveraging a vast database of chemical reactions to predict feasible synthetic routes.
One-Step Synthesis Focus: Specifically designed for one-step synthesis, it provides concise and direct routes for your target compounds, streamlining the synthesis process.
Accurate Predictions: Utilizing the extensive PISTACHIO, BKMS_METABOLIC, PISTACHIO_RINGBREAKER, REAXYS, REAXYS_BIOCATALYSIS database, our tool offers high-accuracy predictions, reflecting the latest in chemical research and data.
Strategy Settings
Precursor scoring | Relevance Heuristic |
---|---|
Min. plausibility | 0.01 |
Model | Template_relevance |
Template Set | Pistachio/Bkms_metabolic/Pistachio_ringbreaker/Reaxys/Reaxys_biocatalysis |
Top-N result to add to graph | 6 |
Feasible Synthetic Routes
試験管内研究製品の免責事項と情報
BenchChemで提示されるすべての記事および製品情報は、情報提供を目的としています。BenchChemで購入可能な製品は、生体外研究のために特別に設計されています。生体外研究は、ラテン語の "in glass" に由来し、生物体の外で行われる実験を指します。これらの製品は医薬品または薬として分類されておらず、FDAから任何の医療状態、病気、または疾患の予防、治療、または治癒のために承認されていません。これらの製品を人間または動物に体内に導入する形態は、法律により厳格に禁止されています。これらのガイドラインに従うことは、研究と実験において法的および倫理的な基準の遵守を確実にするために重要です。