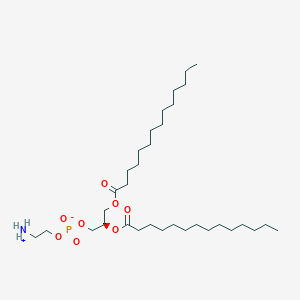
1,2-ジミリストイル-sn-グリセロ-3-ホスホエタノールアミン
概要
説明
1,2-ジミリストイル-sn-グリセロ-3-ホスホエタノールアミンは、生物学的膜に一般的に存在するリン脂質です。これは、グリセロール骨格に結合した2つのミリスチン酸鎖で構成されており、さらにホスホエタノールアミン基に結合しています。 この化合物は、細胞膜の構造的完全性と機能性に重要な役割を果たし、膜の流動性と脂質二重層の形成に寄与しています .
2. 製法
合成経路と反応条件
1,2-ジミリストイル-sn-グリセロ-3-ホスホエタノールアミンは、グリセロールとミリスチン酸のエステル化、続いて得られたジグリセリドのホスホエタノールアミンによるリン酸化によって合成することができます。 反応は一般的に、ジシクロヘキシルカルボジイミド(DCC)やジメチルアミノピリジン(DMAP)などの触媒を用いてエステル化プロセスを促進します .
工業生産方法
1,2-ジミリストイル-sn-グリセロ-3-ホスホエタノールアミンの工業生産には、大規模なエステル化とリン酸化プロセスが関与します。 連続フローリアクターと高度な精製技術を使用することで、最終製品の高収率と高純度が保証されます .
科学的研究の応用
1,2-Dimyristoyl-sn-glycero-3-phosphoethanolamine has a wide range of applications in scientific research:
Chemistry: Used in the synthesis of liposomes and other lipid-based structures for drug delivery systems.
Biology: Plays a role in the study of membrane dynamics and protein-lipid interactions.
Industry: Employed in the production of cosmetics and personal care products due to its emulsifying properties.
作用機序
1,2-ジミリストイル-sn-グリセロ-3-ホスホエタノールアミンは、生物学的膜に組み込まれることで効果を発揮し、膜の流動性と安定性に影響を与えます。それは、膜タンパク質やその他の脂質と相互作用し、それらの機能を調節し、さまざまな細胞プロセスを促進します。 この化合物は、脂質二重層と小胞を形成する能力が、薬物送達と膜研究における役割にとって重要です .
6. 類似の化合物との比較
類似の化合物
1,2-ジミリストイル-sn-グリセロ-3-ホスホコリン: 構造は似ていますが、ホスホエタノールアミンではなくホスホコリン基を含んでいます.
1,2-ジミリストイル-sn-グリセロ-3-ホスホグリセロール: ホスホグリセロール基を含み、同様の用途に使用されます.
1,2-ジミリストイル-sn-グリセロ-3-ホスホエタノールアミン-N-[メトキシ(ポリエチレングリコール)-2000]: 高度な薬物送達システムに使用されるポリエチレングリコールコンジュゲート.
独自性
1,2-ジミリストイル-sn-グリセロ-3-ホスホエタノールアミンは、膜タンパク質との特定の相互作用と、安定な脂質二重層を形成する能力により、独特です。 さまざまな科学的および産業的用途における汎用性は、他の同様の化合物とは一線を画しています .
生化学分析
Biochemical Properties
1,2-Dimyristoyl-sn-glycero-3-phosphoethanolamine plays a significant role in biochemical reactions. It interacts with various enzymes, proteins, and other biomolecules. The nature of these interactions is complex and multifaceted, often involving the formation of lipid bilayers and monolayers .
Cellular Effects
The effects of 1,2-Dimyristoyl-sn-glycero-3-phosphoethanolamine on cells and cellular processes are diverse. It influences cell function by impacting cell signaling pathways, gene expression, and cellular metabolism .
Molecular Mechanism
The molecular mechanism of action of 1,2-Dimyristoyl-sn-glycero-3-phosphoethanolamine involves its interactions at the molecular level. It can bind to biomolecules, inhibit or activate enzymes, and induce changes in gene expression .
Temporal Effects in Laboratory Settings
Over time in laboratory settings, the effects of 1,2-Dimyristoyl-sn-glycero-3-phosphoethanolamine can change. Information on the product’s stability, degradation, and long-term effects on cellular function observed in in vitro or in vivo studies is currently limited .
Metabolic Pathways
1,2-Dimyristoyl-sn-glycero-3-phosphoethanolamine is involved in several metabolic pathways. It interacts with various enzymes and cofactors, and can influence metabolic flux or metabolite levels .
Transport and Distribution
1,2-Dimyristoyl-sn-glycero-3-phosphoethanolamine is transported and distributed within cells and tissues. It can interact with transporters or binding proteins, influencing its localization or accumulation .
Subcellular Localization
The subcellular localization of 1,2-Dimyristoyl-sn-glycero-3-phosphoethanolamine and its effects on activity or function are complex. It may involve targeting signals or post-translational modifications that direct it to specific compartments or organelles .
準備方法
Synthetic Routes and Reaction Conditions
1,2-Dimyristoyl-sn-glycero-3-phosphoethanolamine can be synthesized through the esterification of glycerol with myristic acid, followed by the phosphorylation of the resulting diglyceride with phosphoethanolamine. The reaction typically involves the use of catalysts such as dicyclohexylcarbodiimide (DCC) and dimethylaminopyridine (DMAP) to facilitate the esterification process .
Industrial Production Methods
Industrial production of 1,2-dimyristoyl-sn-glycero-3-phosphoethanolamine involves large-scale esterification and phosphorylation processes. The use of continuous flow reactors and advanced purification techniques ensures high yield and purity of the final product .
化学反応の分析
反応の種類
1,2-ジミリストイル-sn-グリセロ-3-ホスホエタノールアミンは、次のようなさまざまな化学反応を起こします。
酸化: ミリスチン酸鎖は、酸化されてヒドロペルオキシドやその他の酸化生成物を生成することができます。
還元: ホスホエタノールアミン基は、還元されてホスホエタノールアミン誘導体を生成することができます。
一般的な試薬と条件
酸化: 一般的な酸化剤には、過酸化水素や過マンガン酸カリウムなどがあります。
還元: 水素化ホウ素ナトリウムや水素化リチウムアルミニウムなどの還元剤が使用されます。
生成される主な生成物
酸化: ヒドロペルオキシドとアルデヒド。
還元: ホスホエタノールアミン誘導体。
置換: さまざまな置換グリセロール誘導体.
4. 科学研究における用途
1,2-ジミリストイル-sn-グリセロ-3-ホスホエタノールアミンは、科学研究において幅広い用途があります。
類似化合物との比較
Similar Compounds
1,2-Dimyristoyl-sn-glycero-3-phosphocholine: Similar in structure but contains a phosphocholine group instead of phosphoethanolamine.
1,2-Dimyristoyl-sn-glycero-3-phosphoglycerol: Contains a phosphoglycerol group and is used in similar applications.
1,2-Dimyristoyl-sn-glycero-3-phosphoethanolamine-N-[methoxy(polyethylene glycol)-2000]: A polyethylene glycol conjugate used in advanced drug delivery systems.
Uniqueness
1,2-Dimyristoyl-sn-glycero-3-phosphoethanolamine is unique due to its specific interaction with membrane proteins and its ability to form stable lipid bilayers. Its versatility in various scientific and industrial applications sets it apart from other similar compounds .
特性
CAS番号 |
998-07-2 |
---|---|
分子式 |
C33H66NO8P |
分子量 |
635.9 g/mol |
IUPAC名 |
2-azaniumylethyl [(2R)-2,3-di(tetradecanoyloxy)propyl] phosphate |
InChI |
InChI=1S/C33H66NO8P/c1-3-5-7-9-11-13-15-17-19-21-23-25-32(35)39-29-31(30-41-43(37,38)40-28-27-34)42-33(36)26-24-22-20-18-16-14-12-10-8-6-4-2/h31H,3-30,34H2,1-2H3,(H,37,38)/t31-/m1/s1 |
InChIキー |
NEZDNQCXEZDCBI-WJOKGBTCSA-N |
SMILES |
CCCCCCCCCCCCCC(=O)OCC(COP(=O)([O-])OCC[NH3+])OC(=O)CCCCCCCCCCCCC |
異性体SMILES |
CCCCCCCCCCCCCC(=O)OC[C@H](COP(=O)([O-])OCC[NH3+])OC(=O)CCCCCCCCCCCCC |
正規SMILES |
CCCCCCCCCCCCCC(=O)OCC(COP(=O)([O-])OCC[NH3+])OC(=O)CCCCCCCCCCCCC |
外観 |
Unit:100 mgSolvent:nonePurity:98+%Physical solid |
Key on ui other cas no. |
998-07-2 |
物理的記述 |
Solid |
同義語 |
1,2-dimyristoyl-sn-glycero-3-phosphoethanolamine 1,2-dimyristoylphosphatidylethanolamine 1,2-ditetradecanoyl-sn-glycero-3-phosphoethanolamine dimyristoyl cephalin DMPE tetradecanoic acid, (1R)-1-((((2-aminoethoxy)hydroxyphosphinyl)oxy)methyl)-1,2-ethanediyl este |
製品の起源 |
United States |
Retrosynthesis Analysis
AI-Powered Synthesis Planning: Our tool employs the Template_relevance Pistachio, Template_relevance Bkms_metabolic, Template_relevance Pistachio_ringbreaker, Template_relevance Reaxys, Template_relevance Reaxys_biocatalysis model, leveraging a vast database of chemical reactions to predict feasible synthetic routes.
One-Step Synthesis Focus: Specifically designed for one-step synthesis, it provides concise and direct routes for your target compounds, streamlining the synthesis process.
Accurate Predictions: Utilizing the extensive PISTACHIO, BKMS_METABOLIC, PISTACHIO_RINGBREAKER, REAXYS, REAXYS_BIOCATALYSIS database, our tool offers high-accuracy predictions, reflecting the latest in chemical research and data.
Strategy Settings
Precursor scoring | Relevance Heuristic |
---|---|
Min. plausibility | 0.01 |
Model | Template_relevance |
Template Set | Pistachio/Bkms_metabolic/Pistachio_ringbreaker/Reaxys/Reaxys_biocatalysis |
Top-N result to add to graph | 6 |
Feasible Synthetic Routes
Q1: How does 1,2-Dimyristoyl-sn-glycero-3-phosphoethanolamine (DMPE) interact with antimicrobial peptides (AMPs)?
A: AMPs can insert themselves into DMPE bilayers to varying degrees, influencing the dynamics of the phospholipids. [] The interaction appears to be stronger with DMPE than with phosphatidylcholine (PC) lipids, leading to increased destabilization and morphological changes in DMPE vesicles upon AMP addition. []
Q2: What is the role of DMPE in bacterial membranes and its interaction with host defense peptides?
A: DMPE is a significant component of bacterial membranes. Research indicates that some host defense peptides, like Maximin H5, exhibit a higher binding affinity for DMPE-rich membranes. [] This interaction can limit the peptide's ability to lyse the bacterial membrane, potentially contributing to bacterial resistance against host defense mechanisms. []
Q3: Can the interaction between DMPE and cholesterol impact membrane properties?
A: Yes, nearest-neighbor recognition experiments suggest that cholesterol's condensing effect on phospholipid bilayers is primarily observed in the liquid-ordered phase. [] This implies that the presence of cholesterol can significantly influence the packing and organization of DMPE molecules within the membrane. []
Q4: What is the molecular formula and weight of DMPE?
A4: The molecular formula of DMPE is C36H72NO8P, and its molecular weight is 637.9 g/mol.
Q5: How does the chain length of phospholipids in a mixture affect the permeability of the resulting bilayers?
A: Studies using liposomes made from mixtures of DMPE and 1,2-distearoyl-sn-glycero-3-phosphocholine (DSPC) show that the permeability of the bilayer is affected by the composition of the lipid phases at the boundaries between liquid crystal and gel states. [] This suggests that DMPE may segregate at these boundaries, influencing the overall permeability of the membrane. []
Q6: How does DMPE behave in mixed monolayers with other phospholipids?
A: DMPE exhibits different miscibility behaviors with various phospholipids. While it shows limited miscibility with phosphoglycerol (PG)-like lipids in the fluid bilayer state, [] it displays non-ideal mixing behavior with 1,2-distearoyl-sn-glycero-3-phosphoethanolamine (DSPE) in the presence of certain diluents like 1,2-dilauroyl-sn-glycero-3-phosphocholine (DLPC). []
Q7: What is the impact of temperature on the organization of water molecules around DMPE bilayers?
A: Research using Small Angle X-ray Scattering (SAXS) and densitometry revealed that the water layer surrounding DMPE bilayers, particularly the "perturbed water" layer, expands with increasing temperature. [] This indicates a temperature-dependent interaction between DMPE and water molecules at the membrane interface. []
Q8: Does the presence of cholesterol affect the interaction between DMPE and water?
A: Studies utilizing nearest-neighbor recognition experiments with exchangeable DMPE dimers suggest that replacing water (H2O) with deuterium oxide (D2O) impacts DMPE mixing only in saturated bilayers lacking cholesterol. [] This indicates that cholesterol may influence the hydration properties of DMPE-containing membranes. []
A8: This section is not applicable as DMPE is primarily a structural lipid and not known to possess inherent catalytic properties.
Q9: How can molecular dynamics simulations be employed to understand DMPE interactions?
A: Molecular dynamics simulations can offer valuable insights into the behavior of DMPE within biological systems. For instance, simulations have been used to examine the stability of myelin basic protein (MBP) on mixed DMPC/DMPE bilayers. [] These simulations revealed that the composition of the lipid bilayer influences MBP's interaction with the membrane, providing insights into the structural dynamics of myelin. []
Q10: Can molecular modeling help understand the interaction of drug conjugates with DMPE membranes?
A: Yes, molecular modeling techniques, in conjunction with experimental methods like Langmuir monolayer studies, can provide insights into how drug conjugates interact with DMPE-based model bacterial membranes. [] This approach can reveal details about the binding modes, conformational changes, and potential impact on membrane hydration. []
Q11: How does the linkage region of sphingolipids influence their mixing with DMPE in cholesterol-rich environments?
A: Nearest-neighbor recognition studies suggest that the linkage region of sphingolipids plays a crucial role in their interaction with phospholipids like DMPE, especially in cholesterol-rich bilayers. [] The presence of cholesterol enhances the preference for homolipid association, indicating that the sphingolipid's linkage region contributes to its segregation from DMPE within the membrane. []
Q12: How does the bridging group in exchangeable DMPE dimers influence nearest-neighbor recognition (NNR) within fluid bilayers?
A: Research indicates that the length and chemical nature of the bridging group in DMPE dimers can significantly impact NNR within fluid bilayers. [] Longer bridging groups tend to enhance NNR, suggesting that they influence the packing and organization of DMPE molecules. [] Cholesterol's effect on NNR was also found to be dependent on the bridging group length. []
Q13: How stable are liposomes composed of DMPE, dihexadecyl phosphate (DCP), and cholesterol?
A: Liposomes with varying ratios of DMPE, DCP, and cholesterol have been studied for their physicochemical properties and biological activity. [] Results suggest that these liposomes are stable at physiological temperatures, making them suitable for in vivo applications. [] The addition of DCP appears to improve colloidal stability. []
A13: Specific SHE regulations are not explicitly discussed in the provided research papers.
A13: The provided research focuses mainly on the biophysical properties and interactions of DMPE rather than its pharmacokinetic and pharmacodynamic aspects.
Q14: How effective are liposomes containing DMPE in delivering therapeutic agents?
A: Studies have investigated the efficacy of DMPE-containing liposomes as delivery systems for therapeutic agents. Liposomes prepared with DMPE and DCP showed the best efficiency in an in vitro model mimicking in vivo release of bioactive substances. []
Q15: How does the presence of DMPE in bacterial membranes contribute to bacterial resistance?
A: The presence of DMPE in bacterial membranes can contribute to resistance against certain antimicrobial agents, such as host defense peptides. [] The specific interaction between DMPE and these peptides can hinder their membrane-disrupting activity, allowing bacteria to evade the immune response. []
A15: Toxicity and safety data specific to DMPE are not explicitly detailed in the provided research papers.
Q16: Can hyaluronic acid-decorated liposomes be used for targeted drug delivery to the skin?
A: Research suggests that hyaluronic acid-decorated liposomes, particularly those incorporating DMPE as a lipid anchor, hold promise for targeted drug delivery to the skin. [] The deformability of these liposomes and the extent of hyaluronic acid conjugation appear to be crucial factors influencing skin penetration. []
Q17: What is the potential of lipid nanoparticles containing DMPE for siRNA delivery to immune cells?
A: Lipid nanoparticles composed of a novel cationic lipid, DOPE, and PEG2000-DMPE have shown potential for siRNA delivery to immune cells like macrophages and dendritic cells. [] These nanoparticles demonstrated efficient siRNA encapsulation and successful gene knockdown in both in vitro and in vivo models. []
A17: Specific biomarkers and diagnostics related to DMPE are not directly addressed in the provided research papers.
Q18: What are some analytical techniques used to study DMPE-containing systems?
A18: Various techniques have been employed to characterize and study DMPE-containing systems. Some examples include:
- Langmuir monolayer studies: These studies provide insights into the interactions of DMPE with other molecules at the air-water interface. [, , ]
- Atomic force microscopy (AFM): AFM can be used to visualize the morphology and organization of DMPE molecules within model membranes. []
- Neutron reflectometry: This technique helps determine the structure and thickness of DMPE-containing layers within multilayered films. []
- Quasi-elastic neutron scattering (QENS): QENS provides information about the dynamics of DMPE molecules and associated water molecules within bilayers. []
- Polarization modulation infrared reflection-absorption spectroscopy (PM-IRRAS): PM-IRRAS offers insights into the orientation and conformation of DMPE molecules within monolayers. []
- Brewster angle microscopy (BAM): BAM visualizes the morphology and phase behavior of DMPE monolayers at the air-water interface. [, ]
- Differential scanning calorimetry (DSC): DSC measures the thermal transitions of DMPE-containing systems, providing insights into their phase behavior. [, , ]
- Dynamic light scattering (DLS): DLS measures the size and diffusion properties of DMPE-containing liposomes and vesicles. [, , , ]
- Small angle X-ray/neutron scattering (SAXS/SANS): SAXS and SANS provide structural information about DMPE-containing bilayers and vesicles. [, , ]
- Time-resolved small-angle neutron scattering (TR-SANS): TR-SANS investigates the dynamics of lipid flip-flop and exchange within DMPE-containing vesicles. [, ]
試験管内研究製品の免責事項と情報
BenchChemで提示されるすべての記事および製品情報は、情報提供を目的としています。BenchChemで購入可能な製品は、生体外研究のために特別に設計されています。生体外研究は、ラテン語の "in glass" に由来し、生物体の外で行われる実験を指します。これらの製品は医薬品または薬として分類されておらず、FDAから任何の医療状態、病気、または疾患の予防、治療、または治癒のために承認されていません。これらの製品を人間または動物に体内に導入する形態は、法律により厳格に禁止されています。これらのガイドラインに従うことは、研究と実験において法的および倫理的な基準の遵守を確実にするために重要です。