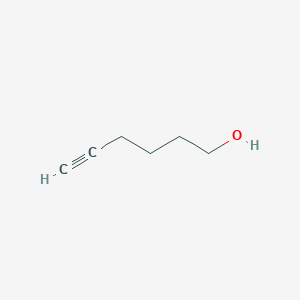
5-ヘキシン-1-オール
説明
5-Hexyn-1-ol, also known as 1-Hexyn-6-ol or 1-Hydroxy-5-hexyne, is an organic compound with the molecular formula C₆H₁₀O. It is a colorless to pale yellow liquid that is slightly miscible with water. This compound is characterized by the presence of both an alkyne group and a hydroxyl group, making it a versatile intermediate in organic synthesis .
科学的研究の応用
5-Hexyn-1-ol is widely used in scientific research due to its versatility:
Chemistry: It serves as a building block for the synthesis of complex organic molecules, including cinnoline-fused cyclic enediynes and lycopodium alkaloids.
Biology: The compound is used in the synthesis of biologically active molecules, which can be studied for their potential therapeutic effects.
Medicine: Research into 5-Hexyn-1-ol derivatives has shown potential in developing new pharmaceuticals.
Industry: It is used in the production of specialty chemicals and materials, including polymers and resins.
作用機序
Target of Action
5-Hexyn-1-ol is a chemical compound with the linear formula HC≡C(CH2)4OH . The primary target of 5-Hexyn-1-ol is the respiratory system . .
Mode of Action
It is known that 5-hexyn-1-ol can be used as a starting material for the preparation of terminal alkynes and lactones containing terminal alkyne groups .
Biochemical Pathways
5-Hexyn-1-ol has been used in the synthesis of various compounds, including cinnoline-fused cyclic enediyne, lycopodium alkaloids, (+)-nankakurine A and (+)-nankakurine B, and 7-benzoyloxy-3-(2-nitrophenylseleno)-1,5-cyclodecadiyne
Pharmacokinetics
Its physical properties such as boiling point (73-75 °c/15 mmhg) and density (089 g/mL at 25 °C) suggest that it may have certain bioavailability characteristics .
Result of Action
It is known that 5-hexyn-1-ol can cause irritation to the skin, eyes, and respiratory system .
Action Environment
Environmental factors can influence the action, efficacy, and stability of 5-Hexyn-1-ol. For instance, it is a flammable liquid and should be kept away from open flames and ignition sources . It also has a green odor and is soluble in water . These characteristics suggest that the compound’s action may be influenced by factors such as temperature, humidity, and the presence of other chemical substances.
準備方法
Synthetic Routes and Reaction Conditions: 5-Hexyn-1-ol can be synthesized through various methods. One common method involves the reaction of 1-bromo-5-chloropentane with sodium acetylide, followed by hydrolysis. Another method includes the reduction of 5-hexynoic acid using lithium aluminum hydride.
Industrial Production Methods: In industrial settings, 5-Hexyn-1-ol is often produced through the catalytic hydration of 1-hexyne. This process involves the use of a palladium catalyst and a suitable solvent under controlled temperature and pressure conditions .
Types of Reactions:
Oxidation: 5-Hexyn-1-ol can undergo oxidation to form 5-hexynoic acid. This reaction typically uses oxidizing agents such as potassium permanganate or chromium trioxide.
Reduction: The compound can be reduced to 5-hexyn-1-amine using reducing agents like lithium aluminum hydride.
Substitution: 5-Hexyn-1-ol can participate in nucleophilic substitution reactions, where the hydroxyl group is replaced by other functional groups.
Common Reagents and Conditions:
Oxidation: Potassium permanganate, chromium trioxide
Reduction: Lithium aluminum hydride
Substitution: Various nucleophiles under basic or acidic conditions
Major Products:
Oxidation: 5-Hexynoic acid
Reduction: 5-Hexyn-1-amine
Substitution: Various substituted hexynes depending on the nucleophile used
類似化合物との比較
- 4-Pentyn-1-ol
- 3-Butyn-1-ol
- 5-Hexynoic acid
- 4-Pentynoic acid
- 5-Hexen-1-ol
- 2-Hexyn-1-ol
Comparison: 5-Hexyn-1-ol is unique due to its specific combination of an alkyne and a hydroxyl group, which provides it with distinct reactivity compared to similar compounds. For instance, 4-Pentyn-1-ol and 3-Butyn-1-ol have shorter carbon chains, affecting their physical properties and reactivity. 5-Hexynoic acid and 4-Pentynoic acid contain carboxylic acid groups instead of hydroxyl groups, leading to different chemical behaviors .
特性
IUPAC Name |
hex-5-yn-1-ol | |
---|---|---|
Source | PubChem | |
URL | https://pubchem.ncbi.nlm.nih.gov | |
Description | Data deposited in or computed by PubChem | |
InChI |
InChI=1S/C6H10O/c1-2-3-4-5-6-7/h1,7H,3-6H2 | |
Source | PubChem | |
URL | https://pubchem.ncbi.nlm.nih.gov | |
Description | Data deposited in or computed by PubChem | |
InChI Key |
GOQJMMHTSOQIEI-UHFFFAOYSA-N | |
Source | PubChem | |
URL | https://pubchem.ncbi.nlm.nih.gov | |
Description | Data deposited in or computed by PubChem | |
Canonical SMILES |
C#CCCCCO | |
Source | PubChem | |
URL | https://pubchem.ncbi.nlm.nih.gov | |
Description | Data deposited in or computed by PubChem | |
Molecular Formula |
C6H10O | |
Source | PubChem | |
URL | https://pubchem.ncbi.nlm.nih.gov | |
Description | Data deposited in or computed by PubChem | |
DSSTOX Substance ID |
DTXSID70239148 | |
Record name | 5-Hexyn-1-ol | |
Source | EPA DSSTox | |
URL | https://comptox.epa.gov/dashboard/DTXSID70239148 | |
Description | DSSTox provides a high quality public chemistry resource for supporting improved predictive toxicology. | |
Molecular Weight |
98.14 g/mol | |
Source | PubChem | |
URL | https://pubchem.ncbi.nlm.nih.gov | |
Description | Data deposited in or computed by PubChem | |
CAS No. |
928-90-5 | |
Record name | 5-Hexyn-1-ol | |
Source | CAS Common Chemistry | |
URL | https://commonchemistry.cas.org/detail?cas_rn=928-90-5 | |
Description | CAS Common Chemistry is an open community resource for accessing chemical information. Nearly 500,000 chemical substances from CAS REGISTRY cover areas of community interest, including common and frequently regulated chemicals, and those relevant to high school and undergraduate chemistry classes. This chemical information, curated by our expert scientists, is provided in alignment with our mission as a division of the American Chemical Society. | |
Explanation | The data from CAS Common Chemistry is provided under a CC-BY-NC 4.0 license, unless otherwise stated. | |
Record name | 5-Hexyn-1-ol | |
Source | ChemIDplus | |
URL | https://pubchem.ncbi.nlm.nih.gov/substance/?source=chemidplus&sourceid=0000928905 | |
Description | ChemIDplus is a free, web search system that provides access to the structure and nomenclature authority files used for the identification of chemical substances cited in National Library of Medicine (NLM) databases, including the TOXNET system. | |
Record name | 5-Hexyn-1-ol | |
Source | EPA DSSTox | |
URL | https://comptox.epa.gov/dashboard/DTXSID70239148 | |
Description | DSSTox provides a high quality public chemistry resource for supporting improved predictive toxicology. | |
Record name | 5-Hexyn-1-ol | |
Source | European Chemicals Agency (ECHA) | |
URL | https://echa.europa.eu/substance-information/-/substanceinfo/100.131.698 | |
Description | The European Chemicals Agency (ECHA) is an agency of the European Union which is the driving force among regulatory authorities in implementing the EU's groundbreaking chemicals legislation for the benefit of human health and the environment as well as for innovation and competitiveness. | |
Explanation | Use of the information, documents and data from the ECHA website is subject to the terms and conditions of this Legal Notice, and subject to other binding limitations provided for under applicable law, the information, documents and data made available on the ECHA website may be reproduced, distributed and/or used, totally or in part, for non-commercial purposes provided that ECHA is acknowledged as the source: "Source: European Chemicals Agency, http://echa.europa.eu/". Such acknowledgement must be included in each copy of the material. ECHA permits and encourages organisations and individuals to create links to the ECHA website under the following cumulative conditions: Links can only be made to webpages that provide a link to the Legal Notice page. | |
Retrosynthesis Analysis
AI-Powered Synthesis Planning: Our tool employs the Template_relevance Pistachio, Template_relevance Bkms_metabolic, Template_relevance Pistachio_ringbreaker, Template_relevance Reaxys, Template_relevance Reaxys_biocatalysis model, leveraging a vast database of chemical reactions to predict feasible synthetic routes.
One-Step Synthesis Focus: Specifically designed for one-step synthesis, it provides concise and direct routes for your target compounds, streamlining the synthesis process.
Accurate Predictions: Utilizing the extensive PISTACHIO, BKMS_METABOLIC, PISTACHIO_RINGBREAKER, REAXYS, REAXYS_BIOCATALYSIS database, our tool offers high-accuracy predictions, reflecting the latest in chemical research and data.
Strategy Settings
Precursor scoring | Relevance Heuristic |
---|---|
Min. plausibility | 0.01 |
Model | Template_relevance |
Template Set | Pistachio/Bkms_metabolic/Pistachio_ringbreaker/Reaxys/Reaxys_biocatalysis |
Top-N result to add to graph | 6 |
Feasible Synthetic Routes
ANone: 5-Hexyn-1-ol is an organic compound with the molecular formula C₆H₁₀O and a molecular weight of 98.14 g/mol. It features both a terminal alkyne group (-C≡CH) at one end and a primary alcohol group (-OH) at the other end of a six-carbon chain.
ANone: 5-Hexyn-1-ol can be characterized using various spectroscopic methods:
ANone: The presence of both the terminal alkyne and the primary alcohol group makes 5-Hexyn-1-ol a versatile building block in organic synthesis.
- Initiator in Polymerization: The hydroxyl group can act as an initiator in ring-opening polymerization reactions, leading to the formation of terminal-functionalized polyesters. []
- Click Chemistry: The alkyne group is a prime target for "click chemistry" reactions, particularly the copper(I)-catalyzed azide-alkyne cycloaddition (CuAAC). This reaction is highly efficient and allows for the introduction of diverse functionalities by reacting 5-Hexyn-1-ol with various azide-containing molecules. [, ]
- Formation of Cyclic Compounds: 5-Hexyn-1-ol can undergo cyclization reactions, often catalyzed by transition metals like gold. For example, it can react with indoles in a gold-catalyzed cascade reaction to form ketone derivatives after an unusual intramolecular redox process. []
A: Yes, 5-Hexyn-1-ol has been utilized in the synthesis of diunsaturated hydroxy acids, which are precursors to macrolide aggregation pheromones found in certain grain beetles. The synthesis involves coupling 5-Hexyn-1-ol with functionalized propargylic bromide, followed by oxidation and selective hydrogenation steps. []
A: Yes, different positional and geometrical isomers of 5-Hexyn-1-ol, as well as the corresponding hexen-1-ols, have been synthesized. These methods often start with reactions using sodium acetylide in liquid ammonia, followed by selective reduction or hydrogenation steps to achieve the desired isomers. []
A: 5-Hexyn-1-ol can participate in free-radical mediated reactions. For instance, in the stannylcarbonylation of hydroxy-substituted alkynes, 5-Hexyn-1-ol yields cyclized carbonylated products, specifically lactols, as the major products. This occurs through the trapping of intermediate acyl radicals by the internal hydroxyl group. []
A: Yes, a hydroxy-functionalized 3,6-bis(2-pyridyl)pyridazine ligand synthesized from 5-Hexyn-1-ol and 3,6-bis(2-pyridyl)tetrazine was used in the construction of polymeric [2 × 2] grids. [] The ligand was incorporated into poly(l-lactide) macroligands, which upon complexation with metal ions, resulted in the formation of supramolecular grid-like architectures.
A: Research indicates that 5-Hexyn-1-ol can inhibit carbon steel corrosion in deaerated solutions containing a mixture of picolinic acid and ascorbic acid. This inhibition likely occurs through chemisorption of 5-Hexyn-1-ol onto the metal surface. The efficiency of inhibition increases with temperature, further supporting the chemical nature of the adsorption process. []
ANone: Absolutely. 5-Hexyn-1-ol plays a significant role in synthesizing various steroid derivatives.
- Diazabicyclo-Steroid Derivatives: 5-Hexyn-1-ol is crucial in the [2+2] cycloaddition with protected testosterone, progesterone, or pregnenolone. These reactions ultimately lead to the formation of diazabicyclo-steroid derivatives, some of which have shown antibacterial activity against Staphylococcus aureus and Streptococcus pneumoniae. []
- Steroid-Oxirane Derivatives: 5-Hexyn-1-ol is employed in the synthesis of steroid-oxirane derivatives through a series of reactions involving its coupling with 2-nitroestrone or 2-nitroestradiol. []
- Dioxa Diazaspiro[bicyclo[9.4.2]Heptadecane-Steroid-Dienyne Derivatives: 5-Hexyn-1-ol is a key starting material in a multistep synthesis of complex steroid derivatives, including a dioxa-diazaspiro[bicyclo[9.4.2]heptadecane-steroid-dienyne derivative. This synthesis highlights the versatility of 5-Hexyn-1-ol in building complex molecular architectures. []
A: Pulse radiolysis studies have investigated the reactions of 5-Hexyn-1-ol with primary species generated from water radiolysis, including hydrated electrons (eaq⁻), hydrogen atoms (H atoms), and hydroxyl radicals (•OH). It was found that 5-Hexyn-1-ol reacts with these species at different rates, leading to the formation of transient species that exhibit reducing properties. [] For instance, the reaction with H atoms produces vinyl-type radicals.
試験管内研究製品の免責事項と情報
BenchChemで提示されるすべての記事および製品情報は、情報提供を目的としています。BenchChemで購入可能な製品は、生体外研究のために特別に設計されています。生体外研究は、ラテン語の "in glass" に由来し、生物体の外で行われる実験を指します。これらの製品は医薬品または薬として分類されておらず、FDAから任何の医療状態、病気、または疾患の予防、治療、または治癒のために承認されていません。これらの製品を人間または動物に体内に導入する形態は、法律により厳格に禁止されています。これらのガイドラインに従うことは、研究と実験において法的および倫理的な基準の遵守を確実にするために重要です。