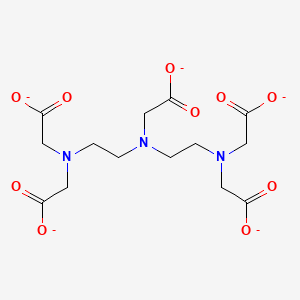
Diethylenetriaminepentaacetate
- 専門家チームからの見積もりを受け取るには、QUICK INQUIRYをクリックしてください。
- 品質商品を競争力のある価格で提供し、研究に集中できます。
説明
Pentetate(5-) is a pentacarboxylic acid anion. It is a conjugate base of a pentetate(4-).
科学的研究の応用
Occupational Health & Safety : DTPA, particularly its pentasodium salt form, is used in the chemical industry as a polymerization initiator. Its toxic properties have been studied, leading to the establishment of safety standards for its use in work environments (Ogudov et al., 2022).
Medical Imaging : DTPA is used as a contrast agent in medical imaging, notably in dynamic renal studies with 99mTc-DTPA. It helps in analyzing kidney functions and structures (Diffey et al., 1976).
Biochemical Research : It has been utilized for covalently coupling to proteins, creating hexadentate chelating sites for metal ions, which are helpful in biological systems' probes (Krejcarek & Tucker, 1977).
Detection and Analysis : DTPA's ability to form a stable complex with lead (II) has been harnessed in developing high-performance liquid chromatographic methods for its detection in biological fluids (Weber et al., 1999).
Radiation Exposure and Toxicology : DTPA is an effective agent in facilitating the elimination of radionuclides like americium and plutonium from the body, as seen in its usage for decorporation of inhaled Am (Sueda et al., 2014).
Environmental Studies : It has been used to study the uptake of metals by plants, with implications for agricultural and environmental science (Wallace, 1972).
Pharmacokinetics : Studies have been conducted on the in vivo dissolution rate and pharmacokinetics of DTPA in various formulations, indicating its potential in drug delivery and medical diagnostics (Daly et al., 1982).
特性
CAS番号 |
14047-41-7 |
---|---|
分子式 |
C14H18N3O10-5 |
分子量 |
388.31 g/mol |
IUPAC名 |
2-[bis[2-[bis(carboxylatomethyl)amino]ethyl]amino]acetate |
InChI |
InChI=1S/C14H23N3O10/c18-10(19)5-15(1-3-16(6-11(20)21)7-12(22)23)2-4-17(8-13(24)25)9-14(26)27/h1-9H2,(H,18,19)(H,20,21)(H,22,23)(H,24,25)(H,26,27)/p-5 |
InChIキー |
QPCDCPDFJACHGM-UHFFFAOYSA-I |
SMILES |
C(CN(CC(=O)[O-])CC(=O)[O-])N(CCN(CC(=O)[O-])CC(=O)[O-])CC(=O)[O-] |
正規SMILES |
C(CN(CC(=O)[O-])CC(=O)[O-])N(CCN(CC(=O)[O-])CC(=O)[O-])CC(=O)[O-] |
14047-41-7 | |
製品の起源 |
United States |
Retrosynthesis Analysis
AI-Powered Synthesis Planning: Our tool employs the Template_relevance Pistachio, Template_relevance Bkms_metabolic, Template_relevance Pistachio_ringbreaker, Template_relevance Reaxys, Template_relevance Reaxys_biocatalysis model, leveraging a vast database of chemical reactions to predict feasible synthetic routes.
One-Step Synthesis Focus: Specifically designed for one-step synthesis, it provides concise and direct routes for your target compounds, streamlining the synthesis process.
Accurate Predictions: Utilizing the extensive PISTACHIO, BKMS_METABOLIC, PISTACHIO_RINGBREAKER, REAXYS, REAXYS_BIOCATALYSIS database, our tool offers high-accuracy predictions, reflecting the latest in chemical research and data.
Strategy Settings
Precursor scoring | Relevance Heuristic |
---|---|
Min. plausibility | 0.01 |
Model | Template_relevance |
Template Set | Pistachio/Bkms_metabolic/Pistachio_ringbreaker/Reaxys/Reaxys_biocatalysis |
Top-N result to add to graph | 6 |
Feasible Synthetic Routes
試験管内研究製品の免責事項と情報
BenchChemで提示されるすべての記事および製品情報は、情報提供を目的としています。BenchChemで購入可能な製品は、生体外研究のために特別に設計されています。生体外研究は、ラテン語の "in glass" に由来し、生物体の外で行われる実験を指します。これらの製品は医薬品または薬として分類されておらず、FDAから任何の医療状態、病気、または疾患の予防、治療、または治癒のために承認されていません。これらの製品を人間または動物に体内に導入する形態は、法律により厳格に禁止されています。これらのガイドラインに従うことは、研究と実験において法的および倫理的な基準の遵守を確実にするために重要です。