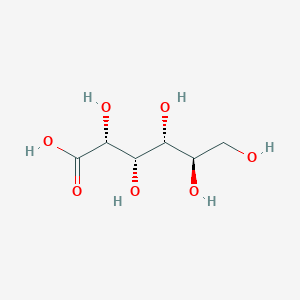
グルコン酸
概要
説明
L-グルコン酸は、分子式がC6H12O7の有機化合物です。中性水溶液ではグルコン酸アニオンを形成する白色の結晶性固体です。この化合物は、果物、蜂蜜、ワインなど、自然界に広く存在しています。 さまざまな生化学的プロセスにおける役割と、さまざまな産業における用途で知られています .
2. 製法
合成経路と反応条件: L-グルコン酸は、通常、グルコースオキシダーゼ酵素の存在下でグルコースを好気的に酸化することにより生成されます。 反応により、グルコンラクトンと過酸化水素が生成され、その後加水分解されてグルコン酸が生成されます . この反応は次のように表すことができます: [ \text{C}6\text{H}{12}\text{O}6 + \text{O}_2 \rightarrow \text{C}6\text{H}{10}\text{O}_6 + \text{H}_2\text{O}_2 ] [ \text{C}6\text{H}{10}\text{O}_6 + \text{H}_2\text{O} \rightarrow \text{C}6\text{H}{12}\text{O}_7 ]
工業的製造方法: L-グルコン酸の工業的製造には、アスペルギルス・ニガーやグルコノバクター・オキシダンスなどの真菌や細菌を用いた微生物発酵が用いられることが多いです。これらの微生物は、制御された条件下でグルコースをグルコン酸に酸化します。 このプロセスはコスト効率が高く、微生物細胞のリサイクルと再利用が可能になります {_svg_3}.
3. 化学反応解析
反応の種類: L-グルコン酸は、以下のようなさまざまな化学反応を起こします。
酸化: グルカル酸にさらに酸化される可能性があります。
還元: グルコンラクトンに還元される可能性があります。
置換: さまざまなカチオンとエステルや塩を形成できます。
一般的な試薬と条件:
酸化: 酸素の存在下で、白金やパラジウムなどの触媒が使用されます。
還元: 金属触媒の存在下での水素ガス。
置換: 塩基と反応してグルコン酸塩を形成します。
主な生成物:
酸化: グルカル酸。
還元: グルコンラクトン。
4. 科学研究における用途
L-グルコン酸は、科学研究や産業において幅広い用途があります。
化学: 金属イオンを結合するためのキレート剤として使用されます。
生物学: 代謝経路において役割を果たし、生化学的アッセイで使用されます。
医学: カルシウム欠乏症の治療のためのグルコン酸カルシウムなどの医薬品の製剤に使用されます。
産業: 食品や飲料では酸度調整剤として、洗浄剤では鉱物沈殿物を溶解するために、建設ではセメントの水和速度を遅くするためのコンクリート混和剤として使用されます
科学的研究の応用
Production Methods
Gluconic acid is primarily produced through microbial fermentation, utilizing various microorganisms such as Aspergillus niger and Gluconobacter species. The fermentation process can employ different carbon sources, including agricultural byproducts, which enhances sustainability and cost-effectiveness. The following table summarizes the primary production methods for gluconic acid:
Production Method | Microorganisms | Substrates Used | Yield |
---|---|---|---|
Fermentation | Aspergillus niger | Corn starch, grape must | High yield |
Enzymatic Catalysis | Glucose oxidase | Glucose | Moderate yield |
Electrochemical Synthesis | Various catalysts | Glucose | Variable yield |
Industrial Applications
Gluconic acid and its derivatives find extensive use in several industries:
- Food Industry : Used as a food additive and flavoring agent, gluconic acid enhances the taste of products like sherbets and can reduce fat absorption in baked goods .
- Pharmaceuticals : GA acts as a chelating agent in drug formulations, improving the solubility and bioavailability of certain medications .
- Construction : Sodium gluconate is utilized as a concrete additive to enhance workability and extend setting time .
- Agriculture : It serves as a biostimulant and chelating agent for micronutrients in fertilizers, improving nutrient uptake by plants .
Health Benefits
Recent studies indicate that gluconic acid may have potential health benefits, particularly in cancer treatment. Research suggests that gluconate can inhibit tumor growth by blocking citrate uptake in cancer cells . Furthermore, GA's role in modulating gut microbiota through metabolomic interactions has been highlighted, showcasing its importance in gut health .
Case Studies
- Microbial Production Optimization : A study demonstrated that using agro-industrial byproducts as substrates for GA production not only reduced costs but also increased yields significantly. This approach utilized Aureobasidium pullulans, resulting in an efficient fermentation process that produced high-quality gluconic acid .
- Application in Food Products : In a controlled trial, the addition of gluconic acid to low-fat doughnut formulations resulted in reduced fat absorption during frying without compromising taste or texture. This application highlights GA's potential to enhance food quality while promoting healthier options .
作用機序
L-グルコン酸は、主に金属イオンをキレート化する能力によって効果を発揮します。さまざまな金属イオンと安定な錯体を形成し、さまざまな生化学的および工業的プロセスで利用できます。 分子標的としては、カルシウム、鉄、その他の金属イオンがあり、関連する経路は金属イオンの輸送と安定化に関連しています .
生化学分析
Biochemical Properties
Gluconic acid is typically produced by the aerobic oxidation of glucose in the presence of the enzyme glucose oxidase . The conversion produces gluconolactone and hydrogen peroxide . The lactone spontaneously hydrolyzes to gluconic acid in water . Gluconic acid is involved in a wide range of cascade reactions .
Cellular Effects
Gluconic acid has been found to influence the composition and activity of the intestinal microflora, which may improve growth performance of piglets after weaning . It enhances performance in period d 0–14 post-weaning, in particular feed intake was increased .
Molecular Mechanism
The chemical structure of gluconic acid consists of a six-carbon chain, with five hydroxyl groups positioned in the same way as in the open-chained form of glucose, terminating in a carboxylic acid group . The oxidation of β-D-glucopyranose by molecular oxygen obeys a ping pong bi–bi kinetic mechanism . For the reductive half-reaction, β-D-glucopyranose is oxidized to D-glucono-δ-lactone by transferring a proton from its C1-hydroxyl to a basic group on the enzyme (His516), and a direct hydride transfer occurs from its C1 position to the N5 position of the isoalloxazine ring of FAD .
Temporal Effects in Laboratory Settings
The expression of the enzyme glucose oxidase, which is involved in the production of gluconic acid, at the transcriptional and translational levels in grape berries increased within 3 weeks after full bloom and then decreased . Almost no transcript of the enzyme was detected in the mature berries, but the enzyme protein was detectable .
Dosage Effects in Animal Models
In piglets, feeding gluconic acid enhanced performance in period d 0–14 post-weaning, in particular feed intake was increased . The high dose did not show benefits over the low dose . Regarding d 0–42, feed intake was elevated .
Metabolic Pathways
Gluconic acid is an aldonic acid derived from ß-D-glucose via a site-specific oxidation, in which the aldehyde group (-CHO) at C-1 is oxidized electrochemically or catalytically into a carboxyl group (-COOH) . It can also be obtained through the hydrolysis of D-gluconolactone .
Transport and Distribution
It is known that gluconic acid is poorly absorbed but readily fermented to butyrate in the gut .
Subcellular Localization
It is known that the enzyme glucose oxidase, which is involved in the production of gluconic acid, is secreted .
準備方法
Synthetic Routes and Reaction Conditions: L-Gluconic acid is typically produced by the aerobic oxidation of glucose in the presence of the enzyme glucose oxidase. The reaction produces gluconolactone and hydrogen peroxide, which subsequently hydrolyzes to form gluconic acid . The reaction can be represented as follows: [ \text{C}6\text{H}{12}\text{O}_6 + \text{O}_2 \rightarrow \text{C}6\text{H}{10}\text{O}_6 + \text{H}_2\text{O}_2 ] [ \text{C}6\text{H}{10}\text{O}_6 + \text{H}_2\text{O} \rightarrow \text{C}6\text{H}{12}\text{O}_7 ]
Industrial Production Methods: Industrial production of L-Gluconic acid often involves microbial fermentation using fungi or bacteria such as Aspergillus niger or Gluconobacter oxydans. These microorganisms oxidize glucose to gluconic acid under controlled conditions. The process is cost-effective and allows for the recycling and reuse of microbial cells .
化学反応の分析
Types of Reactions: L-Gluconic acid undergoes various chemical reactions, including:
Oxidation: It can be further oxidized to glucaric acid.
Reduction: It can be reduced to gluconolactone.
Substitution: It can form esters and salts with different cations.
Common Reagents and Conditions:
Oxidation: Catalysts such as platinum or palladium are used in the presence of oxygen.
Reduction: Hydrogen gas in the presence of a metal catalyst.
Substitution: Reacts with bases to form gluconate salts.
Major Products:
Oxidation: Glucaric acid.
Reduction: Gluconolactone.
Substitution: Various gluconate salts such as calcium gluconate and sodium gluconate.
類似化合物との比較
L-グルコン酸は、次のような他の類似化合物と比較することができます。
グルカル酸: 追加のカルボン酸基を持つ、グルコン酸の酸化型。
グルコンラクトン: グルコン酸のラクトン型で、グルコン酸に再び加水分解される可能性があります。
グルクロン酸: 構造は似ていますが、酸化状態と官能基が異なります。
独自性: L-グルコン酸は、安定なグルコン酸塩を形成する能力と、自然界に広く存在することによって独自です。 金属イオンと錯体を形成する汎用性により、さまざまな用途で価値があります .
生物活性
Gluconic acid, a mild organic acid derived from glucose through enzymatic oxidation, has garnered attention for its diverse biological activities. This article explores the biological effects of gluconic acid, focusing on its antimicrobial properties, potential as a biomarker for health conditions, and its role in plant growth promotion and stress alleviation.
Overview of Gluconic Acid
Gluconic acid (C₆H₁₂O₇) is produced naturally by various microorganisms, including bacteria and fungi. It is recognized for its applications in food, pharmaceuticals, and biotechnology. The production of gluconic acid primarily occurs through the oxidation of glucose facilitated by enzymes such as glucose oxidase and glucose dehydrogenase.
Antimicrobial Activity
Research has demonstrated that gluconic acid exhibits significant antimicrobial properties. A study highlighted the antimicrobial activity of gluconic acid produced by the bacterium Gluconacetobacter diazotrophicus Pal5. This strain showed a broad antagonistic spectrum against both Gram-positive and Gram-negative bacteria, although eukaryotic microorganisms displayed more resistance to inhibition by gluconic acid . The presence of other organic acids can modify this inhibition, indicating a complex interaction within microbial communities.
Table 1: Antimicrobial Spectrum of Gluconic Acid
Microbial Type | Sensitivity to Gluconic Acid |
---|---|
Gram-positive Bacteria | Sensitive |
Gram-negative Bacteria | Sensitive |
Eukaryotic Microorganisms | More Resistant |
Gluconic Acid as a Biomarker
Recent studies have identified gluconic acid as a potential race-specific biomarker for hypertension and stroke risk, particularly in Black adults. An analysis conducted at the International Stroke Conference 2023 indicated that higher levels of gluconic acid were associated with increased risks of ischemic stroke and hypertension among this demographic . The study utilized data from the REGARDS cohort, demonstrating that gluconic acid could mediate significant associations between lifestyle factors and cardiovascular risks.
Key Findings from the REGARDS Study
- Association with Hypertension : Odds ratio (OR) for hypertension in Black adults was 1.86 (95% CI: 1.39-2.47).
- Association with Ischemic Stroke : Hazard ratio (HR) for ischemic stroke was 1.53 (95% CI: 1.28-1.81).
- Mediation Effect : Gluconic acid mediated 25.4% of the association between hypertension and ischemic stroke .
Role in Plant Growth Promotion
Gluconic acid plays a crucial role in plant growth promotion by enhancing nutrient availability through solubilization processes. A study focusing on Pseudomonas fluorescens CHA0 revealed that gluconic acid production was linked to the solubilization of inorganic phosphates, which are vital for plant nutrition . Furthermore, gluconic acid has been shown to alleviate aluminum toxicity in plants, thereby promoting root growth under stressful conditions .
Table 2: Effects of Gluconic Acid on Plant Growth
Parameter | Effect |
---|---|
Phosphate Solubilization | Increased availability |
Aluminum Toxicity Alleviation | Enhanced root growth |
Case Studies on Gluconic Acid Applications
- Microbial Production : Research indicates that microbial fermentation is a favored method for producing gluconic acid using various carbon sources, including glucose hydrolysates .
- Antifungal Properties : Studies have shown that gluconic acid production in Pseudomonas fluorescens contributes to antifungal activity against root pathogens, enhancing crop resilience .
- Health Implications : The identification of gluconic acid as a biomarker opens avenues for improving dietary habits and managing cardiovascular health among specific populations .
特性
CAS番号 |
157663-13-3 |
---|---|
分子式 |
C6H12O7 |
分子量 |
196.16 g/mol |
IUPAC名 |
(2S,3R,4S,5S)-2,3,4,5,6-pentahydroxyhexanoic acid |
InChI |
InChI=1S/C6H12O7/c7-1-2(8)3(9)4(10)5(11)6(12)13/h2-5,7-11H,1H2,(H,12,13)/t2-,3-,4+,5-/m0/s1 |
InChIキー |
RGHNJXZEOKUKBD-KLVWXMOXSA-N |
SMILES |
C(C(C(C(C(C(=O)O)O)O)O)O)O |
異性体SMILES |
C([C@@H]([C@@H]([C@H]([C@@H](C(=O)O)O)O)O)O)O |
正規SMILES |
C(C(C(C(C(C(=O)O)O)O)O)O)O |
Color/Form |
NEEDLES WHITE POWDER NEEDLES FROM ETHANOL & ETHER CRYSTALS |
密度 |
greater than 1 at 68 °F (USCG, 1999) 1.24 @ 25 °C/4 °C 1.23 g/cm³ |
melting_point |
131.0 °C 131 °C 113-118°C |
Key on ui other cas no. |
133-42-6 526-95-4 10361-31-6 |
物理的記述 |
Ammonium gluconate is a white solid with a weak odor of ammonia. Sinks and mixes with water. (USCG, 1999) Liquid Colourless to light yellow, clear syrupy liquid Solid WHITE CRYSTALLINE POWDER. |
ピクトグラム |
Irritant |
関連するCAS |
10101-21-0 (strontium[2:1] salt) 10361-31-6 (unspecified ammonium salt) 13005-35-1 (unspecified copper salt) 14906-97-9 (unspecified hydrochloride salt) 22830-45-1 (unspecified iron(+2) salt dihydrate) 299-27-4 (mono-potassium salt) 35087-77-5 (unspecified potassium salt) 35984-19-1 (unspecified tin(+2) salt) 3632-91-5 (magnesium[2:1] salt) 527-07-1 (mono-hydrochloride salt) 60007-93-4 (aluminum[3:1] salt) 60816-70-8 (mono-lithium salt) 6485-39-8 (manganese[2:1] salt) 82139-35-3 (unspecified zinc salt) |
賞味期限 |
TURNS YELLOW ON EXPOSURE TO LIGHT |
溶解性 |
1.61 M IN WATER: 31.6 G/100 ML @ 25 °C; SLIGHTLY SOL IN ALCOHOL; PRACTICALLY INSOL IN MOST ORGANIC SOLVENTS FREELY SOL IN WATER; SLIGHTLY SOL IN ALC, INSOLUBLE IN ETHER AND MOST OTHER ORGANIC SOLVENTS 316 mg/mL at 25 °C Solubility in water, g/100ml at 25 °C: 100 (good) |
同義語 |
oron gluconate D-gluconate D-gluconic acid dextronic acid gluconate gluconic acid gluconic acid, (113)indium-labeled gluconic acid, (14)C-labeled gluconic acid, (159)dysprosium-labeled salt gluconic acid, (99)technecium (5+) salt gluconic acid, 1-(14)C-labeled gluconic acid, 6-(14)C-labeled gluconic acid, aluminum (3:1) salt gluconic acid, ammonium salt gluconic acid, calcium salt gluconic acid, cesium(+3) salt gluconic acid, cobalt (2:1) salt gluconic acid, copper salt gluconic acid, Fe(+2) salt, dihydrate gluconic acid, lanthanum(+3) salt gluconic acid, magnesium (2:1) salt gluconic acid, manganese (2:1) salt gluconic acid, monolithium salt gluconic acid, monopotassium salt gluconic acid, monosodium salt gluconic acid, potassium salt gluconic acid, sodium salt gluconic acid, strontium (2:1) salt gluconic acid, tin(+2) salt gluconic acid, zinc salt lithium gluconate magnerot magnesium gluconate maltonic acid manganese gluconate pentahydroxycaproic acid sodium gluconate zinc gluconate |
製品の起源 |
United States |
Retrosynthesis Analysis
AI-Powered Synthesis Planning: Our tool employs the Template_relevance Pistachio, Template_relevance Bkms_metabolic, Template_relevance Pistachio_ringbreaker, Template_relevance Reaxys, Template_relevance Reaxys_biocatalysis model, leveraging a vast database of chemical reactions to predict feasible synthetic routes.
One-Step Synthesis Focus: Specifically designed for one-step synthesis, it provides concise and direct routes for your target compounds, streamlining the synthesis process.
Accurate Predictions: Utilizing the extensive PISTACHIO, BKMS_METABOLIC, PISTACHIO_RINGBREAKER, REAXYS, REAXYS_BIOCATALYSIS database, our tool offers high-accuracy predictions, reflecting the latest in chemical research and data.
Strategy Settings
Precursor scoring | Relevance Heuristic |
---|---|
Min. plausibility | 0.01 |
Model | Template_relevance |
Template Set | Pistachio/Bkms_metabolic/Pistachio_ringbreaker/Reaxys/Reaxys_biocatalysis |
Top-N result to add to graph | 6 |
Feasible Synthetic Routes
試験管内研究製品の免責事項と情報
BenchChemで提示されるすべての記事および製品情報は、情報提供を目的としています。BenchChemで購入可能な製品は、生体外研究のために特別に設計されています。生体外研究は、ラテン語の "in glass" に由来し、生物体の外で行われる実験を指します。これらの製品は医薬品または薬として分類されておらず、FDAから任何の医療状態、病気、または疾患の予防、治療、または治癒のために承認されていません。これらの製品を人間または動物に体内に導入する形態は、法律により厳格に禁止されています。これらのガイドラインに従うことは、研究と実験において法的および倫理的な基準の遵守を確実にするために重要です。