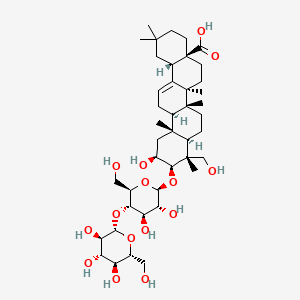
Bayogenin 3-O-cellobioside
- 専門家チームからの見積もりを受け取るには、QUICK INQUIRYをクリックしてください。
- 品質商品を競争力のある価格で提供し、研究に集中できます。
説明
Bayogenin 3-O-cellobioside is a pentacyclic triterpenoid that is bayogenin substituted at the O-3 position by a cellobiosyl residue. It has a role as a plant metabolite. It is a cellobioside, a disaccharide derivative, a pentacyclic triterpenoid, a triterpenoid saponin and a monocarboxylic acid. It derives from a bayogenin.
科学的研究の応用
Plant Defense Mechanism Against Fungi
Bayogenin 3-O-cellobioside has been identified as a crucial compound in the defense mechanisms of rice cultivars against the rice blast fungus, Pyricularia oryzae. A study conducted by Norvienyeku et al. (2020) in the Plant Biotechnology Journal found that this compound levels in rice directly correlated with the plant's resistance attributes. This compound was expressed in both susceptible and resistant rice cultivars during infection, suggesting its role in plant immunity and indicating the potential for engineering saponin expression in cereal crops as a sustainable disease management strategy (Norvienyeku et al., 2020).
Role in Insect Resistance
This compound is also significant in the context of insect resistance. In a study published in the Journal of Chemical Ecology, Nielsen et al. (2010) explored the effects of various saponins, including this compound, on food consumption in different flea beetle lines. The research suggested a relationship between saponin structure and its defensive properties against insects (Nielsen et al., 2010).
Biological Evaluation as Glycogen Phosphorylase Inhibitors
This compound has been evaluated for its potential as a glycogen phosphorylase inhibitor. In a study by Wen et al. (2010) in the Chinese Journal of Natural Medicines, the compound showed moderate inhibitory activity, which is significant for understanding its potential therapeutic applications (Wen et al., 2010).
特性
CAS番号 |
92622-05-4 |
---|---|
分子式 |
C42H68O15 |
分子量 |
813 g/mol |
IUPAC名 |
(4aS,6aR,6aS,6bR,8aR,9R,10R,11S,12aR,14bS)-10-[(2R,3R,4R,5S,6R)-3,4-dihydroxy-6-(hydroxymethyl)-5-[(2S,3R,4S,5S,6R)-3,4,5-trihydroxy-6-(hydroxymethyl)oxan-2-yl]oxyoxan-2-yl]oxy-11-hydroxy-9-(hydroxymethyl)-2,2,6a,6b,9,12a-hexamethyl-1,3,4,5,6,6a,7,8,8a,10,11,12,13,14b-tetradecahydropicene-4a-carboxylic acid |
InChI |
InChI=1S/C42H68O15/c1-37(2)11-13-42(36(52)53)14-12-40(5)20(21(42)15-37)7-8-26-38(3)16-22(46)33(39(4,19-45)25(38)9-10-41(26,40)6)57-35-31(51)29(49)32(24(18-44)55-35)56-34-30(50)28(48)27(47)23(17-43)54-34/h7,21-35,43-51H,8-19H2,1-6H3,(H,52,53)/t21-,22-,23+,24+,25+,26+,27+,28-,29+,30+,31+,32+,33-,34-,35-,38-,39-,40+,41+,42-/m0/s1 |
InChIキー |
GQPGGSOQFNPVJI-XXRVHFCASA-N |
異性体SMILES |
C[C@@]12CC[C@@H]3[C@@]([C@H]1CC=C4[C@]2(CC[C@@]5([C@H]4CC(CC5)(C)C)C(=O)O)C)(C[C@@H]([C@@H]([C@@]3(C)CO)O[C@H]6[C@@H]([C@H]([C@@H]([C@H](O6)CO)O[C@H]7[C@@H]([C@H]([C@@H]([C@H](O7)CO)O)O)O)O)O)O)C |
SMILES |
CC1(CCC2(CCC3(C(=CCC4C3(CCC5C4(CC(C(C5(C)CO)OC6C(C(C(C(O6)CO)OC7C(C(C(C(O7)CO)O)O)O)O)O)O)C)C)C2C1)C)C(=O)O)C |
正規SMILES |
CC1(CCC2(CCC3(C(=CCC4C3(CCC5C4(CC(C(C5(C)CO)OC6C(C(C(C(O6)CO)OC7C(C(C(C(O7)CO)O)O)O)O)O)O)C)C)C2C1)C)C(=O)O)C |
製品の起源 |
United States |
試験管内研究製品の免責事項と情報
BenchChemで提示されるすべての記事および製品情報は、情報提供を目的としています。BenchChemで購入可能な製品は、生体外研究のために特別に設計されています。生体外研究は、ラテン語の "in glass" に由来し、生物体の外で行われる実験を指します。これらの製品は医薬品または薬として分類されておらず、FDAから任何の医療状態、病気、または疾患の予防、治療、または治癒のために承認されていません。これらの製品を人間または動物に体内に導入する形態は、法律により厳格に禁止されています。これらのガイドラインに従うことは、研究と実験において法的および倫理的な基準の遵守を確実にするために重要です。