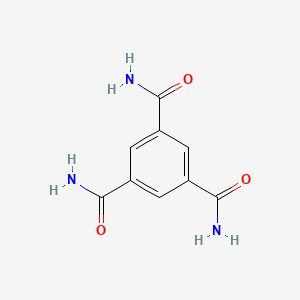
Benzene-1,3,5-tricarboxamide
概要
説明
Benzene-1,3,5-tricarboxamide is a versatile compound known for its simple structure and wide accessibility. First synthesized in 1915 by Curtius, it has become increasingly important in various scientific disciplines due to its supramolecular self-assembly behavior . This compound is a key building block in applications ranging from nanotechnology to polymer processing and biomedical applications .
作用機序
Target of Action
Benzene-1,3,5-tricarboxamide (BTA) is a versatile molecule that primarily targets the formation of supramolecular structures . Its simple structure and wide accessibility, combined with a detailed understanding of its supramolecular self-assembly behavior, allow full utilization of this versatile, supramolecular building block in applications ranging from nanotechnology to polymer processing and biomedical applications .
Mode of Action
The mode of action of BTA is primarily through the self-assembly into one-dimensional, nanometer-sized rod-like structures stabilized by threefold H-bonding . This self-assembly is driven by the multivalent nature of BTAs, which is particularly useful in the biomedical field .
Biochemical Pathways
The biochemical pathways affected by BTAs are related to their ability to form functional soft-materials by virtue of their complementary hydrogen bonding . They are explored as structurally reinforcing supramolecular building blocks in porous coordination polymers .
Pharmacokinetics
Their solubility and self-assembly behavior are critical factors influencing their bioavailability . For instance, inversed amide BTAs (iBTAs) form intermolecular hydrogen bonds and assemble into supramolecular polymers, albeit with a slight decrease in water solubility .
Result of Action
The result of BTA’s action is the formation of supramolecular structures that mimic fibrous structures found in nature . These structures can be used to generate functional multicomponent biomaterials . For instance, BTAs have been used to create scaffolds for cartilage and bone regeneration, targeted therapy for atherosclerosis, and the promotion of angiogenesis .
Action Environment
The action of BTAs can be influenced by environmental factors. For example, the dynamic properties of BTAs can be tuned by mixing mono- and bivalent variants . This allows the control of the number of biological recognition motifs presented on the supramolecular structures by mixing in different functional monomers in the desired ratio .
生化学分析
Biochemical Properties
Benzene-1,3,5-tricarboxamide plays a crucial role in biochemical reactions, particularly in the formation of supramolecular polymers. Its ability to form stable hydrogen bonds and π-stacking interactions allows it to interact with various enzymes, proteins, and other biomolecules. For instance, this compound can interact with serum proteins, which can influence its stability and functionality in biological environments . These interactions are primarily non-covalent, involving hydrogen bonding and hydrophobic interactions, which contribute to the compound’s ability to form complex supramolecular structures .
Cellular Effects
This compound has been shown to influence various cellular processes. It can affect cell signaling pathways, gene expression, and cellular metabolism by interacting with specific proteins and enzymes. For example, studies have demonstrated that this compound can support neuronal outgrowth and cell encapsulation, indicating its potential in tissue engineering and regenerative medicine . Additionally, its ability to form supramolecular polymers can create a biomimetic environment that supports cell viability and function .
Molecular Mechanism
The molecular mechanism of this compound involves its ability to form stable supramolecular assemblies through non-covalent interactions such as hydrogen bonding, π-stacking, and hydrophobic interactions . These interactions enable this compound to self-assemble into one-dimensional nanostructures, which can influence various biochemical and cellular processes.
Temporal Effects in Laboratory Settings
In laboratory settings, the effects of this compound can change over time due to its stability and degradation properties. Studies have shown that this compound supramolecular polymers exhibit a trade-off between stability and responsiveness, which can influence their performance in biological environments . The compound’s stability can be affected by factors such as dilution and protein interactions, which can lead to disassembly and changes in its functionality over time .
Dosage Effects in Animal Models
The effects of this compound can vary with different dosages in animal models. Higher doses of the compound may lead to toxic or adverse effects, while lower doses may be more beneficial for specific applications. For instance, studies have shown that this compound can support cell viability and function at optimal concentrations, but excessive amounts may disrupt cellular processes and lead to negative outcomes .
Metabolic Pathways
This compound is involved in various metabolic pathways, interacting with enzymes and cofactors that influence its metabolic flux and metabolite levels. The compound’s ability to form stable hydrogen bonds and π-stacking interactions allows it to participate in complex biochemical reactions, which can impact its metabolic behavior and overall functionality in biological systems .
Transport and Distribution
The transport and distribution of this compound within cells and tissues are influenced by its interactions with transporters and binding proteins. These interactions can affect the compound’s localization and accumulation in specific cellular compartments, which can impact its activity and function . The ability of this compound to form supramolecular assemblies also plays a role in its transport and distribution within biological systems .
Subcellular Localization
This compound exhibits specific subcellular localization patterns, which can influence its activity and function. The compound’s ability to form stable supramolecular assemblies allows it to target specific compartments or organelles within cells. This targeting can be mediated by post-translational modifications or specific targeting signals that direct this compound to its desired location . The compound’s localization can impact its interactions with other biomolecules and its overall functionality in cellular processes .
準備方法
Synthetic Routes and Reaction Conditions: Benzene-1,3,5-tricarboxamide can be synthesized starting from commercially available 5-aminoisophthalic acid. This approach eliminates the need for desymmetrization of the core, reducing the number of synthetic steps and improving yields . The reaction typically involves the formation of amide bonds through condensation reactions under controlled conditions.
Industrial Production Methods: In industrial settings, the synthesis of this compound often involves the use of high-throughput methods to ensure scalability and efficiency. The process may include the use of automated reactors and continuous flow systems to maintain consistent reaction conditions and high purity of the final product .
化学反応の分析
Types of Reactions: Benzene-1,3,5-tricarboxamide undergoes various chemical reactions, including:
Oxidation: This reaction can be facilitated by strong oxidizing agents, leading to the formation of carboxylic acids.
Reduction: Reduction reactions typically involve the use of reducing agents like lithium aluminum hydride, resulting in the formation of amines.
Common Reagents and Conditions:
Oxidation: Potassium permanganate or chromium trioxide in acidic conditions.
Reduction: Lithium aluminum hydride in anhydrous ether.
Substitution: Nucleophiles such as amines or alcohols under basic conditions.
Major Products:
Oxidation: Carboxylic acids.
Reduction: Amines.
Substitution: Functionalized this compound derivatives.
科学的研究の応用
Benzene-1,3,5-tricarboxamide has a wide range of applications in scientific research:
類似化合物との比較
Benzene-1,3,5-tricarboxylic acid: Similar in structure but lacks the amide groups, leading to different chemical properties and applications.
Benzene-1,3,5-tricarboxamide derivatives: These compounds have varied side arm functionalities, which can significantly affect their self-assembly behavior and applications.
Uniqueness: this compound is unique due to its ability to form strong hydrogen bonds and its versatility in various applications. Its simple structure allows for easy modification, making it a valuable building block in supramolecular chemistry .
特性
IUPAC Name |
benzene-1,3,5-tricarboxamide | |
---|---|---|
Source | PubChem | |
URL | https://pubchem.ncbi.nlm.nih.gov | |
Description | Data deposited in or computed by PubChem | |
InChI |
InChI=1S/C9H9N3O3/c10-7(13)4-1-5(8(11)14)3-6(2-4)9(12)15/h1-3H,(H2,10,13)(H2,11,14)(H2,12,15) | |
Source | PubChem | |
URL | https://pubchem.ncbi.nlm.nih.gov | |
Description | Data deposited in or computed by PubChem | |
InChI Key |
WXWQVSOHWXJBDF-UHFFFAOYSA-N | |
Source | PubChem | |
URL | https://pubchem.ncbi.nlm.nih.gov | |
Description | Data deposited in or computed by PubChem | |
Canonical SMILES |
C1=C(C=C(C=C1C(=O)N)C(=O)N)C(=O)N | |
Source | PubChem | |
URL | https://pubchem.ncbi.nlm.nih.gov | |
Description | Data deposited in or computed by PubChem | |
Molecular Formula |
C9H9N3O3 | |
Source | PubChem | |
URL | https://pubchem.ncbi.nlm.nih.gov | |
Description | Data deposited in or computed by PubChem | |
DSSTOX Substance ID |
DTXSID90332281 | |
Record name | 1,3,5-Benzenetricarboxamide | |
Source | EPA DSSTox | |
URL | https://comptox.epa.gov/dashboard/DTXSID90332281 | |
Description | DSSTox provides a high quality public chemistry resource for supporting improved predictive toxicology. | |
Molecular Weight |
207.19 g/mol | |
Source | PubChem | |
URL | https://pubchem.ncbi.nlm.nih.gov | |
Description | Data deposited in or computed by PubChem | |
CAS No. |
60541-32-4 | |
Record name | 1,3,5-Benzenetricarboxamide | |
Source | CAS Common Chemistry | |
URL | https://commonchemistry.cas.org/detail?cas_rn=60541-32-4 | |
Description | CAS Common Chemistry is an open community resource for accessing chemical information. Nearly 500,000 chemical substances from CAS REGISTRY cover areas of community interest, including common and frequently regulated chemicals, and those relevant to high school and undergraduate chemistry classes. This chemical information, curated by our expert scientists, is provided in alignment with our mission as a division of the American Chemical Society. | |
Explanation | The data from CAS Common Chemistry is provided under a CC-BY-NC 4.0 license, unless otherwise stated. | |
Record name | 1,3,5-Benzenetricarboxamide | |
Source | EPA DSSTox | |
URL | https://comptox.epa.gov/dashboard/DTXSID90332281 | |
Description | DSSTox provides a high quality public chemistry resource for supporting improved predictive toxicology. | |
Retrosynthesis Analysis
AI-Powered Synthesis Planning: Our tool employs the Template_relevance Pistachio, Template_relevance Bkms_metabolic, Template_relevance Pistachio_ringbreaker, Template_relevance Reaxys, Template_relevance Reaxys_biocatalysis model, leveraging a vast database of chemical reactions to predict feasible synthetic routes.
One-Step Synthesis Focus: Specifically designed for one-step synthesis, it provides concise and direct routes for your target compounds, streamlining the synthesis process.
Accurate Predictions: Utilizing the extensive PISTACHIO, BKMS_METABOLIC, PISTACHIO_RINGBREAKER, REAXYS, REAXYS_BIOCATALYSIS database, our tool offers high-accuracy predictions, reflecting the latest in chemical research and data.
Strategy Settings
Precursor scoring | Relevance Heuristic |
---|---|
Min. plausibility | 0.01 |
Model | Template_relevance |
Template Set | Pistachio/Bkms_metabolic/Pistachio_ringbreaker/Reaxys/Reaxys_biocatalysis |
Top-N result to add to graph | 6 |
Feasible Synthetic Routes
Q1: What is the primary driving force for BTA self-assembly?
A: The primary driving force behind BTA self-assembly is the formation of strong, directional threefold α-helical type intermolecular hydrogen bonds between adjacent BTA molecules. [, , ] This leads to the formation of one-dimensional columnar aggregates, often described as helical stacks or nanorods. [, , , , ]
Q2: How does the chirality of BTA monomers influence the self-assembly process?
A: Introducing a stereogenic center into the alkyl side chains of BTAs leads to a preference for one helical conformation over the other, evident in the strong Cotton effects observed in dilute apolar solutions. [, , , ] This chiral amplification arises from the energetic preference of homochiral interactions over heterochiral ones during assembly.
Q3: What types of higher-order structures can BTAs form?
A3: Beyond one-dimensional aggregates, BTAs can assemble into more complex architectures. For instance, they can form:
- Twisted nanofibers: Driven by interfiber interactions and influenced by the peptide sequence in BTA-peptide conjugates. []
- Organogels: Formed in various nonpolar solvents, exhibiting viscoelastic properties dependent on BTA concentration and solvent type. [, , ]
- Membranes and nanotubes: Observed with carboxylic acid functionalized, water-soluble BTAs, showcasing the system's ability to adopt polymorphic structures. []
Q4: How does solvent polarity affect BTA self-assembly?
A: BTA self-assembly is highly sensitive to solvent polarity. Increasing the solvent polarity disrupts the hydrogen bonding network, leading to a decrease in aggregate stability. [, ] This effect is observed both in dilute solutions and in solid-state materials where BTAs are incorporated into polymers. []
Q5: How do the alkyl side chains of BTAs impact their self-assembly?
A5: The length and branching of alkyl side chains significantly impact BTA self-assembly:
- Chain Length: Increasing the chain length generally enhances the stability of aggregates due to stronger van der Waals interactions between side chains. []
- Branching: Branching can influence the packing arrangements and liquid crystalline behavior. For example, branched 3,7-dimethyloctanoyl chains promote the formation of liquid crystalline phases, while linear chains might favor crystalline structures. []
Q6: Can the hydrogen bonding pattern in BTAs be modified?
A: Yes, modifications to the BTA core can alter the hydrogen bonding pattern. For instance, amino ester-based BTAs exhibit both amide-amide (AA) and amide-carboxylate (AC) hydrogen bonding. [] While AC dimers are more thermodynamically stable, AA interactions are crucial for the formation of extended supramolecular polymers. []
Q7: What are some notable properties of BTA-based materials?
A7: BTA-based materials exhibit a range of interesting properties, including:
- Thermotropic liquid crystallinity: Observed in several BTA derivatives, with the mesophase stability and range influenced by side chain structure and intermolecular interactions. [, ]
- Viscoelasticity: BTA organogels demonstrate viscoelastic behavior, with properties tunable by varying BTA concentration, solvent, and temperature. [, ]
- Polar switching: Hydrogen-bonded hexagonal columnar liquid crystal phases formed by BTAs can be aligned by electric fields, displaying polar switching behavior with potential applications in electronic devices. []
- Fluorescence: BTA derivatives incorporating fluorophores like cyanostilbene exhibit aggregation-induced emission enhancement (AIEE), with fluorescence properties tunable by controlling the self-assembly process. []
Q8: What are the potential applications of BTAs in materials science?
A8: The unique self-assembly and stimuli-responsive properties of BTAs make them attractive candidates for various applications, including:
- Electro-optic devices: BTAs that form liquid crystalline phases with polar switching behavior show promise for use in displays, sensors, and other electro-optic applications. [, ]
- Organogels and soft materials: BTA-based organogels, with their tunable viscoelastic properties, can be used in areas like drug delivery, cosmetics, and sensors. [, ]
- Biomimetic materials: The fibrous nature of BTA assemblies and their ability to incorporate biomolecules make them promising for developing biocompatible scaffolds for tissue engineering and drug delivery. [, ]
Q9: How have computational methods been used to study BTA self-assembly?
A9: Computational chemistry plays a crucial role in understanding and predicting BTA self-assembly. Techniques like:
- Density functional theory (DFT): Used to investigate the strength and geometry of hydrogen bonding interactions, providing insights into the driving forces behind BTA self-assembly and the impact of structural modifications. [, , , ]
- Molecular dynamics (MD) simulations: Employed to simulate the self-assembly process in realistic solvents, providing information on aggregate stability, dynamics, and the influence of solvent on assembly. [, , ]
Q10: What is the significance of the cooperativity factor (σ) in BTA self-assembly?
A: The cooperativity factor quantifies the extent of cooperativity in the self-assembly process. BTAs exhibit a wide range of σ values, from isodesmic (σ = 1) to highly cooperative (σ < 10-6). [] Understanding the relationship between BTA structure and σ allows for the rational design of BTA derivatives with tailored self-assembly properties. []
Q11: How does the amide connectivity in BTAs influence their properties?
A: The amide connectivity significantly affects BTA self-assembly. While conventionally synthesized BTAs have carbonyl (C=O)-centered amides, N-centered BTAs (N-BTAs) show weaker aggregation and less pronounced chiral amplification. [] DFT calculations revealed that this difference stems from the lower interaction energy between monomeric units in N-BTA supramolecular polymers. []
試験管内研究製品の免責事項と情報
BenchChemで提示されるすべての記事および製品情報は、情報提供を目的としています。BenchChemで購入可能な製品は、生体外研究のために特別に設計されています。生体外研究は、ラテン語の "in glass" に由来し、生物体の外で行われる実験を指します。これらの製品は医薬品または薬として分類されておらず、FDAから任何の医療状態、病気、または疾患の予防、治療、または治癒のために承認されていません。これらの製品を人間または動物に体内に導入する形態は、法律により厳格に禁止されています。これらのガイドラインに従うことは、研究と実験において法的および倫理的な基準の遵守を確実にするために重要です。