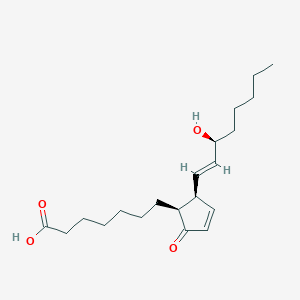
8-iso-PGA1
概要
説明
8-iso-Prostaglandin A1 (8-iso-PGA1) is a cyclopentenone isoprostane derived from non-enzymatic oxidation of arachidonic acid under oxidative stress. It shares structural similarities with prostaglandins (PGs) but differs in stereochemical configuration and biological activity. Its molecular formula is C₂₀H₃₂O₄, with a molecular weight of 336.466 g/mol, and it adopts an (8β,13E,15S) configuration . This compound is recognized for its roles in redox signaling, modulation of Ras proteins, and association with metabolic disorders like hypertension and type 2 diabetes .
準備方法
Synthetic Routes and Reaction Conditions
8-iso-Prostaglandin A1 can be synthesized through the peroxidation of arachidonic acid. This process involves the use of free radicals and reactive oxygen species to induce the formation of isoprostanes. The reaction typically requires specific conditions such as controlled temperature and the presence of catalysts to ensure the desired product is obtained .
Industrial Production Methods
Industrial production of 8-iso-Prostaglandin A1 is not widely documented, but it generally follows similar principles to laboratory synthesis. The process involves large-scale peroxidation reactions, often using advanced techniques to control the reaction environment and optimize yield .
化学反応の分析
Chemical Reactions of 8-iso-PGA1
8-iso-PGA1 participates in several key reactions relevant to its biological function:
-
Enzymatic Reactions It acts as a substrate for specific enzymes such as the Arabidopsis thaliana 12-oxophytodienoate reductases. In these reactions, 8-iso-Prostaglandin A1 undergoes reductions that alter its structural conformation and reactivity.
-
Reactions with Oxidoreductases It can act as a substrate for specific enzymes such as the Arabidopsis thaliana 12-oxophytodienoate reductases. In these reactions, 8-iso-Prostaglandin A1 undergoes reductions that alter its structural conformation and reactivity.
Interactions with Proteins and Receptors
The compound's structural features allow it to engage in specific binding interactions that modulate enzymatic activity and influence cellular signaling pathways. For instance, studies have shown that its cyclopentenone structure facilitates interactions with histidine residues in enzymes, impacting substrate specificity and catalytic efficiency.
Isomerization
-
The addition of β-cyclodextrins (CDs) promoted the isomerization to 8-iso . In kinetic studies in aqueous solution, Limaprost was degraded to 17S,20-dimethyl-trans-Δ(2)-PGA1 (11-deoxy-Δ(10)) and 17S,20-dimethyl-trans-Δ(2)-8-iso-PGE1 (8-iso) .
Biological Activities
8-iso-Prostaglandin A1 exhibits significant biological activities, including the modulation of neurotransmitter release. Research indicates that it inhibits potassium-induced release of D-aspartate from isolated bovine retinas, suggesting a role in neuroprotection and modulation of synaptic activity. Furthermore, as an isoprostane, it is implicated in various physiological processes, including inflammation and vascular homeostasis.
AtOPR3 Interactions
The crystal structure of Arabidopsis thaliana OPR3 in complex with 8-iso prostaglandin A1 (8-iso PGA 1) which has the same α,β-unsaturated carbonyl group and stereochemistry in the cyclopentenone ring as in the physiologically relevant 9 S,13 S-OPDA .
科学的研究の応用
Biomarker for Oxidative Stress
8-iso-PGA1 is recognized as a significant biomarker for oxidative stress, particularly in human health and disease contexts. Research indicates that the levels of 8-iso-PGF2α, a related compound, correlate with oxidative damage and various diseases. Notably, studies have shown that the ratio of 8-iso-PGF2α to PGF2α can effectively distinguish between different synthesis pathways of these biomarkers, providing insights into oxidative stress mechanisms in vivo .
Case Study: Clinical Relevance
A systematic review involving nearly 900 human studies has established that elevated levels of 8-iso-PGF2α are associated with conditions such as cardiovascular diseases, diabetes, and neurodegenerative disorders. However, it is crucial to interpret these levels carefully, as they may not directly reflect oxidative stress but rather the underlying biochemical pathways involved .
Interaction with Enzymes
This compound interacts specifically with aldo-keto reductase (AKR) enzymes, particularly AKR1B10. This interaction has significant implications for cancer treatment. Studies have demonstrated that this compound inhibits AKR1B10 activity, which is crucial for drug metabolism and resistance in cancer cells. The inhibition leads to increased accumulation of chemotherapeutic agents like doxorubicin, enhancing their efficacy against tumors .
Data Table: Enzymatic Activity Inhibition
Compound | Enzyme Target | Effect on Enzyme Activity | IC50 (µM) |
---|---|---|---|
This compound | AKR1B10 | Inhibition | 22 |
PGA1-B | AKR1B10 | Covalent modification | - |
Structural Studies and Mechanistic Insights
The crystal structure of Arabidopsis thaliana OPR3 in complex with this compound has provided insights into its binding mechanisms and enzymatic activity. The study revealed that this compound stabilizes the substrate molecule for reduction within the enzyme's active site, showcasing its potential as a substrate for various OPR isoforms .
Key Findings from Structural Analysis
- Binding Orientation: The unique binding orientation of this compound suggests relaxed stereospecificity compared to other substrates.
- Enzymatic Activity: The apparent kinetic parameters for the reduction of this compound by AtOPR3 were determined to be and , indicating its viability as an enzymatic substrate .
Antioxidant Properties
Recent investigations have identified this compound as a potential antioxidant agent. Its derivatives have been evaluated for their ability to scavenge free radicals and reduce oxidative damage in various biological systems. The presence of these compounds in biological fluids, such as plasma from cows during stress conditions, highlights their physiological relevance .
Case Study: Antioxidant Evaluation
In bovine models, concentrations of this compound were measured during oxidative stress events. The results indicated a notable increase in antioxidant activity correlated with the presence of these isoprostanes, suggesting their protective roles against oxidative damage .
作用機序
8-iso-Prostaglandin A1 exerts its effects through various molecular targets and pathways. It is known to inhibit aldo-keto reductase family 1 member B10, which plays a role in cellular metabolism and detoxification. The compound also affects potassium-induced neurotransmitter release, indicating its involvement in neural signaling pathways .
類似化合物との比較
Comparison with Structurally Similar Compounds
Structural Isomerism and Chromatographic Behavior
8-iso-PGA1 is a structural isomer of PGA1, differing in the orientation of the hydroxyl and alkyl side chains on the cyclopentenone ring. This isomerism leads to distinct physicochemical properties:
- Retention Time : In liquid chromatography, this compound elutes earlier (16.46 min ) compared to PGA1 (17.59 min ) and PGB1 (17.95 min ), reflecting reduced hydrophobicity due to altered side-chain interactions .
- Mass Spectrometry : Both this compound and PGA1 share the same theoretical mass (335.22169 m/z ), but measured masses differ slightly due to structural variations (Δ = −0.84 ppm for this compound vs. −1.40 ppm for PGA1 ) .
Table 1: Chromatographic and Mass Spectral Data
Compound | Retention Time (min) | Theoretical m/z | Measured m/z | Difference (ppm) |
---|---|---|---|---|
This compound | 16.46 | 335.22169 | 335.22141 | −0.84 |
PGA1 | 17.59 | 335.22169 | 335.22122 | −1.40 |
PGB1 | 17.95 | 335.22169 | 335.22128 | −1.22 |
Enzymatic Interactions and Substrate Specificity
OPR Isoforms and Stereospecificity
Key differences include:
- Active Site Geometry : AtOPR3 has a wider substrate-binding cavity due to Phe74 and His245 residues, whereas AtOPR1’s narrower cavity (with Tyr78 and Tyr246 ) restricts access to bulkier substrates .
- Catalytic Interactions: The cyclopentenone ring of this compound forms stacking interactions with FMN and hydrogen bonds with catalytic residues (His186, His189, Tyr191), positioning it optimally for redox chemistry. In contrast, (9R,13R)-OPDA in LeOPR1 adopts a distinct orientation, with its alkyl chains interacting distally with residues like Arg367 .
Table 2: Enzyme-Substrate Interactions
Enzyme | Substrate | Key Residues | Catalytic Efficiency |
---|---|---|---|
AtOPR3 | This compound | Phe74, His245, Tyr191 | High |
LeOPR1 | (9R,13R)-OPDA | Tyr78, Tyr246, Arg367 | Moderate |
Inhibition of Aldo-Keto Reductases (AKRs)
This compound and its isomers exhibit weaker inhibition of AKR1B10 compared to non-isomerized PGs:
- Trans-Orientation Effect : The trans configuration of this compound’s side chains reduces binding affinity, as shown by IC₅₀ values >43 μM for this compound vs. ~20 μM for PGA1 .
Functional Comparisons in Disease Pathways
Modulation of H-Ras Signaling
This compound covalently modifies Cys118 in the GTP-binding motif of H-Ras, promoting activation of Ras-dependent pathways. In contrast, 15d-PGJ2 and Δ12-PGJ2 preferentially target the C-terminal region (C181/C184 ) .
Table 3: Ras Protein Binding Specificity
Compound | Target Site on H-Ras | Functional Outcome |
---|---|---|
This compound | Cys118 | Ras activation |
15d-PGJ2 | C181/C184 | Altered membrane localization |
Metabolic and Clinical Relevance
- Type 2 Diabetes: this compound is a robust biomarker in a three-eicosanoid risk score, predicting diabetes incidence independently of traditional risk factors (OR >2 for highest vs. lowest quartile) .
- Hypertension : Elevated this compound levels correlate with systolic blood pressure in large cohorts (FINRISK, Framingham) .
- Antipsychotic Effects : Olanzapine treatment reduces this compound levels in mice, linking it to metabolic side effects like weight gain .
生物活性
8-iso-Prostaglandin A1 (8-iso-PGA1) is a bioactive lipid compound that is structurally related to prostaglandins, which are known for their roles in various physiological processes. This compound has garnered attention due to its potential biological activities, particularly in the context of oxidative stress, inflammation, and cancer biology. The understanding of its biological activity is critical for exploring its therapeutic implications.
Chemical Structure and Formation
This compound is derived from arachidonic acid through enzymatic and non-enzymatic pathways. Its formation can occur via the action of cyclooxygenase enzymes (COX-1 and COX-2) or through lipid peroxidation processes. The unique stereochemistry of this compound distinguishes it from other prostaglandins, influencing its biological interactions and activities.
Table 1: Comparison of Prostaglandin Structures
Compound | Structure Type | Formation Pathway |
---|---|---|
Prostaglandin A1 | Enzymatic | COX pathway |
This compound | Non-enzymatic/Enzymatic | Lipid peroxidation/COX pathway |
Oxidative Stress Biomarker
Research indicates that this compound serves as a significant biomarker for oxidative stress. It has been extensively studied in both animal models and human populations, revealing correlations with various diseases, including cardiovascular diseases and cancer. The levels of this compound in biological fluids are often used to assess the extent of oxidative damage.
Case Study: Correlation with Disease
A study involving patients with coronary artery disease demonstrated elevated levels of this compound compared to healthy controls, suggesting its role as a marker for oxidative stress in cardiovascular conditions .
Anti-inflammatory Properties
This compound exhibits anti-inflammatory effects by modulating the activity of various immune cells. It can inhibit the production of pro-inflammatory cytokines, thereby contributing to reduced inflammation in tissues.
Research Findings
In vitro studies have shown that this compound can suppress the expression of TNF-alpha and IL-6 in macrophages, indicating its potential therapeutic role in inflammatory diseases .
Antitumor Activity
Recent investigations have explored the antitumor properties of this compound. It has been shown to inhibit tumor cell proliferation and induce apoptosis in various cancer cell lines.
Table 2: Antitumor Effects of this compound
Cancer Type | Effect Observed | Reference |
---|---|---|
Breast Cancer | Inhibition of cell growth | |
Colorectal Cancer | Induction of apoptosis | |
Lung Cancer | Cell cycle arrest |
The mechanism by which this compound exerts its biological effects involves interaction with specific receptors and modulation of signaling pathways. It is believed to activate peroxisome proliferator-activated receptors (PPARs), which play a crucial role in regulating gene expression related to metabolism, inflammation, and cell growth.
Enzymatic Interactions
Studies have identified that this compound interacts with enzymes such as Arabidopsis thaliana OPR3, demonstrating its substrate specificity and catalytic efficiency . The kinetic parameters established for these interactions indicate that this compound is an effective substrate for certain oxidoreductases.
Q & A
Basic Research Questions
Q. What standardized methodologies are recommended for quantifying 8-iso-PGA1 in biological samples?
- Methodological Answer : Liquid chromatography-tandem mass spectrometry (LC-MS/MS) is the gold standard for quantifying this compound due to its sensitivity and specificity. Protocols include derivatization steps to stabilize the compound and internal standards (e.g., deuterated analogs) for calibration. For example, in bovine models, LC-MS/MS revealed significant concentration shifts during the transition to the early dry period, with this compound levels decreasing from 0.47 nM to baseline over 18 days .
Q. How is this compound implicated in oxidative stress pathways?
- Methodological Answer : this compound is an isoprostane formed via non-enzymatic peroxidation of arachidonic acid, serving as a biomarker of lipid peroxidation. Experimental models (e.g., bovine in vitro oxidative stress assays) demonstrate its elevation under pro-oxidant conditions like AAPH treatment. Researchers should pair this compound measurements with other oxidative markers (e.g., glutathione levels) to contextualize its role in redox imbalance .
Q. What experimental models are suitable for studying this compound in vivo?
- Methodological Answer : Transitional dairy cow models are widely used to study this compound dynamics due to their metabolic stress during the dry period. Longitudinal sampling (e.g., blood or urine) at intervals (e.g., -6 to +12 days relative to calving) captures temporal trends. For mechanistic insights, murine knockout models targeting arachidonate peroxidation pathways (e.g., COX-independent synthesis) are recommended .
Advanced Research Questions
Q. How can structural biology techniques resolve substrate specificity of this compound in enzyme binding?
- Methodological Answer : X-ray crystallography of enzyme-substrate complexes (e.g., Arabidopsis thaliana 12-oxophytodienoate reductase isoform 3 [AtOPR3]) reveals stereospecific interactions. Structural alignment with homologous enzymes (e.g., LeOPR1) highlights conserved residues (e.g., Tyr191 and FMN cofactor) critical for binding. Enzymatic assays (NADPH-dependent A340 monitoring) further validate kinetic parameters, showing AtOPR3’s preferential activity toward this compound over other isoforms (e.g., AtOPR1/2) .
Q. How should researchers address contradictory findings regarding this compound’s association with metabolic outcomes?
- Methodological Answer : Contradictions (e.g., weak correlations with insulin resistance in some cohorts vs. strong associations in others) may arise from population heterogeneity or analytical variability. Mitigation strategies include:
- Multi-cohort validation : Replicate findings in independent datasets (e.g., Framingham Heart Study).
- Adjustment for confounders : Control for age, sex, and obesity in statistical models.
- Pathway analysis : Integrate this compound with upstream/downstream mediators (e.g., 12-HHTrE) to identify network-level effects .
Q. What experimental designs optimize the detection of this compound’s role in cross-species comparative studies?
- Methodological Answer : Use phylogenetically diverse models (e.g., plants, mammals) to assess conserved vs. species-specific functions. For example:
- In plants: Arabidopsis mutants with disrupted jasmonate biosynthesis can clarify this compound’s role in stress signaling.
- In mammals: Compare tissue-specific expression (e.g., liver vs. adipose) using targeted metabolomics. Sedimentation equilibrium assays (e.g., AtOPR3 at 4.2–17.5 k rpm) ensure protein stability across conditions .
Q. How can researchers differentiate this compound’s direct biological effects from confounding oxidative byproducts?
- Methodological Answer : Employ genetic or pharmacological inhibition of peroxidation pathways (e.g., antioxidants like PMG in bovine models) to isolate this compound-specific effects. Pair this with siRNA knockdown of putative receptors (e.g., PPARγ) to dissect signaling mechanisms. Statistical analyses should include sensitivity testing for co-measured isoprostanes (e.g., 8-iso-PGA2) to rule out cross-reactivity .
Q. Methodological Frameworks
- For Experimental Design : Apply the FINER criteria (Feasible, Interesting, Novel, Ethical, Relevant) to prioritize hypotheses. For example, a study on this compound’s role in diabetes should ensure cohort accessibility and translational relevance .
- For Data Interpretation : Use the PICO framework (Population, Intervention, Comparison, Outcome) to structure meta-analyses. For instance, compare this compound levels in oxidative stress interventions (e.g., antioxidant diets) vs. controls .
特性
IUPAC Name |
7-[(1S,2S)-2-[(E,3S)-3-hydroxyoct-1-enyl]-5-oxocyclopent-3-en-1-yl]heptanoic acid | |
---|---|---|
Source | PubChem | |
URL | https://pubchem.ncbi.nlm.nih.gov | |
Description | Data deposited in or computed by PubChem | |
InChI |
InChI=1S/C20H32O4/c1-2-3-6-9-17(21)14-12-16-13-15-19(22)18(16)10-7-4-5-8-11-20(23)24/h12-18,21H,2-11H2,1H3,(H,23,24)/b14-12+/t16-,17-,18-/m0/s1 | |
Source | PubChem | |
URL | https://pubchem.ncbi.nlm.nih.gov | |
Description | Data deposited in or computed by PubChem | |
InChI Key |
BGKHCLZFGPIKKU-DRSVPBQLSA-N | |
Source | PubChem | |
URL | https://pubchem.ncbi.nlm.nih.gov | |
Description | Data deposited in or computed by PubChem | |
Canonical SMILES |
CCCCCC(C=CC1C=CC(=O)C1CCCCCCC(=O)O)O | |
Source | PubChem | |
URL | https://pubchem.ncbi.nlm.nih.gov | |
Description | Data deposited in or computed by PubChem | |
Isomeric SMILES |
CCCCC[C@@H](/C=C/[C@H]1C=CC(=O)[C@H]1CCCCCCC(=O)O)O | |
Source | PubChem | |
URL | https://pubchem.ncbi.nlm.nih.gov | |
Description | Data deposited in or computed by PubChem | |
Molecular Formula |
C20H32O4 | |
Source | PubChem | |
URL | https://pubchem.ncbi.nlm.nih.gov | |
Description | Data deposited in or computed by PubChem | |
Molecular Weight |
336.5 g/mol | |
Source | PubChem | |
URL | https://pubchem.ncbi.nlm.nih.gov | |
Description | Data deposited in or computed by PubChem | |
Physical Description |
Solid | |
Record name | 8-iso-PGA1 | |
Source | Human Metabolome Database (HMDB) | |
URL | http://www.hmdb.ca/metabolites/HMDB0002236 | |
Description | The Human Metabolome Database (HMDB) is a freely available electronic database containing detailed information about small molecule metabolites found in the human body. | |
Explanation | HMDB is offered to the public as a freely available resource. Use and re-distribution of the data, in whole or in part, for commercial purposes requires explicit permission of the authors and explicit acknowledgment of the source material (HMDB) and the original publication (see the HMDB citing page). We ask that users who download significant portions of the database cite the HMDB paper in any resulting publications. | |
Retrosynthesis Analysis
AI-Powered Synthesis Planning: Our tool employs the Template_relevance Pistachio, Template_relevance Bkms_metabolic, Template_relevance Pistachio_ringbreaker, Template_relevance Reaxys, Template_relevance Reaxys_biocatalysis model, leveraging a vast database of chemical reactions to predict feasible synthetic routes.
One-Step Synthesis Focus: Specifically designed for one-step synthesis, it provides concise and direct routes for your target compounds, streamlining the synthesis process.
Accurate Predictions: Utilizing the extensive PISTACHIO, BKMS_METABOLIC, PISTACHIO_RINGBREAKER, REAXYS, REAXYS_BIOCATALYSIS database, our tool offers high-accuracy predictions, reflecting the latest in chemical research and data.
Strategy Settings
Precursor scoring | Relevance Heuristic |
---|---|
Min. plausibility | 0.01 |
Model | Template_relevance |
Template Set | Pistachio/Bkms_metabolic/Pistachio_ringbreaker/Reaxys/Reaxys_biocatalysis |
Top-N result to add to graph | 6 |
Feasible Synthetic Routes
試験管内研究製品の免責事項と情報
BenchChemで提示されるすべての記事および製品情報は、情報提供を目的としています。BenchChemで購入可能な製品は、生体外研究のために特別に設計されています。生体外研究は、ラテン語の "in glass" に由来し、生物体の外で行われる実験を指します。これらの製品は医薬品または薬として分類されておらず、FDAから任何の医療状態、病気、または疾患の予防、治療、または治癒のために承認されていません。これらの製品を人間または動物に体内に導入する形態は、法律により厳格に禁止されています。これらのガイドラインに従うことは、研究と実験において法的および倫理的な基準の遵守を確実にするために重要です。