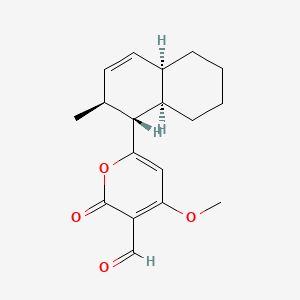
Solanapyrone A
- 専門家チームからの見積もりを受け取るには、QUICK INQUIRYをクリックしてください。
- 品質商品を競争力のある価格で提供し、研究に集中できます。
説明
Solanapyrone A is a solanapyrone, a pyrancarbaldehyde and a member of octahydronaphthalenes. It has a role as an EC 2.7.7.7 (DNA-directed DNA polymerase) inhibitor.
科学的研究の応用
Agricultural Applications
1.1. Pathogenicity and Virulence Factor
Solanapyrone A has been identified as a significant virulence factor in the fungal pathogen Ascochyta rabiei, which affects chickpeas (Cicer arietinum). Research indicates that it contributes to the pathogen's ability to cause disease, making it a target for developing resistant plant varieties. The biosynthesis of this compound involves a gene cluster that encodes enzymes critical for its production, such as polyketide synthases and cytochrome P450 monooxygenases .
1.2. Phytotoxicity
The compound exhibits phytotoxic effects on various plants, particularly chickpeas. Studies have shown that this compound can inhibit plant growth and development, which is an essential consideration in managing crop diseases caused by A. rabiei and similar pathogens .
Pharmacological Applications
2.1. Antifungal Activity
This compound has demonstrated antifungal properties against several pathogenic fungi, including Aspergillus flavus and Fusarium verticillioides. This activity suggests potential applications in developing antifungal agents for agricultural and clinical use .
2.2. Antibacterial Properties
In addition to its antifungal effects, this compound has shown activity against bacterial strains such as Staphylococcus aureus and Candida albicans. This broad-spectrum antimicrobial activity highlights its potential as a therapeutic agent in treating infections caused by these pathogens .
Biochemical Research
3.1. Biosynthetic Studies
Research into the biosynthesis of this compound has led to significant insights into polyketide synthesis pathways. The identification of the gene cluster responsible for its production has paved the way for genetic engineering approaches to enhance or inhibit its synthesis in various fungal species .
3.2. Enzymatic Reactions
This compound's synthesis involves unique enzymatic reactions, including Diels-Alder cycloaddition processes catalyzed by specific enzymes identified in fungal systems. Understanding these mechanisms could facilitate the development of novel synthetic pathways for producing similar compounds .
Case Study: this compound in Fungal Pathogenicity
Table: Biological Activities of this compound
Activity Type | Organism Tested | Result |
---|---|---|
Antifungal | Aspergillus flavus | Effective inhibition |
Antifungal | Fusarium verticillioides | Effective inhibition |
Antibacterial | Staphylococcus aureus | Effective inhibition |
Antibacterial | Candida albicans | Effective inhibition |
Q & A
Basic Research Questions
Q. What are the primary biosynthetic pathways for Solanapyrone A production in fungal species?
this compound is synthesized via a polyketide pathway in fungi such as Ascochyta rabiei. Key genes (e.g., sol4 and sol5) encode enzymes like solanapyrone synthase (sol5) and a transcription factor (sol4) critical for toxin biosynthesis. Gene deletion studies show that sol4 regulates precursor production (prosolanapyrone II), while sol5 catalyzes the final toxin assembly. Mutants lacking these genes fail to produce this compound, confirming their role in biosynthesis .
Q. How does this compound interact with DNA polymerases at a biochemical level?
Kinetic assays using rat DNA polymerase β (pol β) demonstrate that this compound acts as a competitive inhibitor by binding to the enzyme’s DNA template-binding site. Double reciprocal plots revealed increased Kₘ values for DNA templates (147–588% increase) and decreased Kₘ for dNTP substrates (3.05 to 15 pmol/h) in the presence of this compound. Dixon plots calculated inhibition constants (Kᵢ) of 6.8 μM (DNA template) and 22 μM (dNTP substrate), indicating stronger competition with DNA .
Q. What safety precautions are recommended for handling this compound in laboratory settings?
this compound is classified as acutely toxic (oral, dermal) and a respiratory irritant. Researchers must use PPE (gloves, goggles, lab coats), avoid aerosol generation, and ensure proper ventilation. Emergency protocols include immediate eye rinsing and decontamination of exposed skin. Stability data suggest storage in cool, dry conditions away from incompatible materials .
Advanced Research Questions
Q. How can genetic knockouts resolve contradictions in this compound’s role in fungal pathogenesis?
While sol4 and sol5 deletion mutants in A. rabiei abolish toxin production, pathogenicity assays on chickpea seedlings show no reduction in virulence compared to wild-type strains. This contradicts assumptions that this compound is essential for infection. Researchers should contextualize these findings by testing mutants in varied environmental conditions (e.g., stress triggers) or alternative host models to uncover compensatory virulence mechanisms .
Q. What experimental designs are optimal for assessing this compound’s inhibition kinetics?
Use purified DNA polymerases (e.g., pol β) with template-primer systems like poly(dA)/oligo(dT) and dTTP substrates. Conduct Lineweaver-Burk plots to determine Vₘₐₓ and Kₘ under varying inhibitor concentrations. Validate competitive inhibition via Dixon plots and compare binding affinities to structural analogs. Include controls with non-competitive inhibitors (e.g., ddTTP) to confirm specificity .
Q. How can researchers ensure reproducibility in this compound studies?
Document all experimental parameters: enzyme purity (e.g., 0.05 units of pol β per assay), substrate concentrations, and inhibitor storage conditions. Publish raw kinetic data (e.g., pmol/h activity curves) and statistical analyses in supplementary materials. Cross-validate findings using orthogonal methods like surface plasmon resonance (SPR) to measure direct binding to polymerase domains .
Q. What methodologies confirm this compound’s molecular targets in vivo?
Combine genetic knockouts with metabolomic profiling (LC-MS) to track prosolanapyrone II accumulation in sol5 mutants. Use fluorescence polarization assays to assess this compound’s displacement of DNA from polymerase binding sites. In plant models, apply localized toxin delivery via microsyringe and monitor lesion development relative to wild-type infections .
Q. How do structural modifications of this compound affect its bioactivity?
Synthesize analogs with altered pyrone ring substituents and test inhibition constants (Kᵢ) against pol β. Molecular docking simulations can predict binding affinity changes in the 8-kDa N-terminal domain of the polymerase. Correlate structural variations with cytotoxicity assays on chickpea cell cultures to identify structure-activity relationships .
Q. Methodological and Reporting Standards
Q. What criteria define a robust research question for this compound studies?
Focus on unresolved mechanistic gaps (e.g., “How does sol4 regulate prosolanapyrone II synthesis?”) or contradictory findings (e.g., toxin non-essentiality in pathogenesis). Ensure questions are testable via hypothesis-driven experiments (e.g., mutagenesis, kinetic assays) and align with literature on fungal secondary metabolites .
Q. How should researchers address conflicting data on this compound’s ecological role?
Perform meta-analyses of published studies to identify variables influencing toxicity (e.g., host species, fungal strain diversity). Use dual RNA-seq during infection to correlate toxin production with host defense gene expression. Transparently report limitations (e.g., lab vs. field conditions) in discussion sections .
Q. What are the best practices for publishing this compound research?
Follow journal guidelines (e.g., Beilstein Journal of Organic Chemistry):
- Methods : Detail enzyme sources, inhibitor purity (HPLC ≥95%), and assay conditions.
- Data : Include raw kinetic plots (Fig. 3-style graphs) and mutant genotyping protocols.
- Reproducibility : Share supplementary files (e.g., primer sequences for sol gene deletions).
- Ethics : Disclose funding sources and conflicts of interest .
特性
CAS番号 |
88899-61-0 |
---|---|
分子式 |
C18H22O4 |
分子量 |
302.4 g/mol |
IUPAC名 |
6-[(1R,2S,4aR,8aR)-2-methyl-1,2,4a,5,6,7,8,8a-octahydronaphthalen-1-yl]-4-methoxy-2-oxopyran-3-carbaldehyde |
InChI |
InChI=1S/C18H22O4/c1-11-7-8-12-5-3-4-6-13(12)17(11)16-9-15(21-2)14(10-19)18(20)22-16/h7-13,17H,3-6H2,1-2H3/t11-,12+,13+,17+/m0/s1 |
InChIキー |
AWQLNKJBXASXDU-SFDCBXKLSA-N |
SMILES |
CC1C=CC2CCCCC2C1C3=CC(=C(C(=O)O3)C=O)OC |
異性体SMILES |
C[C@H]1C=C[C@H]2CCCC[C@H]2[C@@H]1C3=CC(=C(C(=O)O3)C=O)OC |
正規SMILES |
CC1C=CC2CCCCC2C1C3=CC(=C(C(=O)O3)C=O)OC |
同義語 |
solanapyrone A solanapyrone A, (1R-(1alpha,2alpha,4abeta,8aalpha))-isomer solanapyrone D |
製品の起源 |
United States |
Retrosynthesis Analysis
AI-Powered Synthesis Planning: Our tool employs the Template_relevance Pistachio, Template_relevance Bkms_metabolic, Template_relevance Pistachio_ringbreaker, Template_relevance Reaxys, Template_relevance Reaxys_biocatalysis model, leveraging a vast database of chemical reactions to predict feasible synthetic routes.
One-Step Synthesis Focus: Specifically designed for one-step synthesis, it provides concise and direct routes for your target compounds, streamlining the synthesis process.
Accurate Predictions: Utilizing the extensive PISTACHIO, BKMS_METABOLIC, PISTACHIO_RINGBREAKER, REAXYS, REAXYS_BIOCATALYSIS database, our tool offers high-accuracy predictions, reflecting the latest in chemical research and data.
Strategy Settings
Precursor scoring | Relevance Heuristic |
---|---|
Min. plausibility | 0.01 |
Model | Template_relevance |
Template Set | Pistachio/Bkms_metabolic/Pistachio_ringbreaker/Reaxys/Reaxys_biocatalysis |
Top-N result to add to graph | 6 |
Feasible Synthetic Routes
試験管内研究製品の免責事項と情報
BenchChemで提示されるすべての記事および製品情報は、情報提供を目的としています。BenchChemで購入可能な製品は、生体外研究のために特別に設計されています。生体外研究は、ラテン語の "in glass" に由来し、生物体の外で行われる実験を指します。これらの製品は医薬品または薬として分類されておらず、FDAから任何の医療状態、病気、または疾患の予防、治療、または治癒のために承認されていません。これらの製品を人間または動物に体内に導入する形態は、法律により厳格に禁止されています。これらのガイドラインに従うことは、研究と実験において法的および倫理的な基準の遵守を確実にするために重要です。