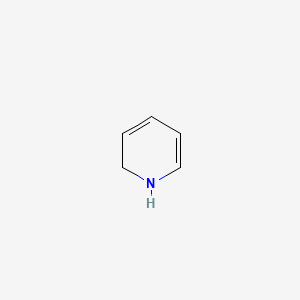
Dihydropyridine
- 専門家チームからの見積もりを受け取るには、QUICK INQUIRYをクリックしてください。
- 品質商品を競争力のある価格で提供し、研究に集中できます。
概要
説明
ジヒドロピリジンは、ピリジン環系を基にした化合物のクラスであり、ピリジン環系は窒素原子を1つ含む6員環です。これらの化合物は、多様な治療用途を持つため、生物学および医学の分野で重要な役割を果たしています。 ジヒドロピリジンは、主にカルシウムチャネルブロッカーとしての役割で知られており、高血圧や狭心症などのさまざまな心臓血管疾患の治療に使用されます .
準備方法
合成ルートと反応条件
ジヒドロピリジンを合成する古典的な方法は、ハントシュピリジン合成です。この多成分反応には、アルデヒド、1,3-ジカルボニル化合物、およびアンモニアの環状縮合が含まれます。 この反応は通常、穏やかな条件下で行われ、収率と選択性を向上させるためにさまざまな試薬で触媒することができます .
工業的生産方法
工業環境では、ジヒドロピリジンの合成には、多くの場合、環境に優しい不均一系触媒が使用されます。これらの触媒は、磁性、シリカ、およびジルコニウムベースのシステムなどの触媒であり、グリーンな条件下で反応を促進し、プロセスをより持続可能かつ費用効果の高いものにします。 ワンポット多成分反応の使用は一般的であり、ジヒドロピリジン誘導体を効率的に生産することができます .
化学反応の分析
反応の種類
ジヒドロピリジンは、酸化、還元、および置換を含むさまざまな化学反応を起こします。これらの反応は、化合物の構造を変更し、生物活性を高めるために不可欠です。
一般的な試薬と条件
酸化: ジヒドロピリジンは、過マンガン酸カリウムまたは過酸化水素などの酸化剤を使用してピリジンに酸化することができます。
還元: ジヒドロピリジンの還元は、水素化ホウ素ナトリウムまたは水素化リチウムアルミニウムなどの還元剤を使用して達成することができます。
置換: 置換反応は、多くの場合、アミンまたはアルコールなどの求核剤を伴い、ジヒドロピリジン環上の水素原子を置き換えます.
生成される主要な生成物
これらの反応から生成される主要な生成物には、さまざまな置換ジヒドロピリジンとピリジンが含まれ、これらは生物活性と治療の可能性を高めています .
科学研究への応用
ジヒドロピリジンは、化学、生物学、医学、および産業の分野で、科学研究において幅広い用途を持っています。注目すべき用途のいくつかを以下に示します。
科学的研究の応用
Dihydropyridines have a wide range of applications in scientific research, particularly in the fields of chemistry, biology, medicine, and industry. Some of the notable applications include:
Chemistry: Dihydropyridines are used as building blocks for the synthesis of more complex molecules.
Biology: In biological research, dihydropyridines are used to study calcium channel function and regulation.
Medicine: Dihydropyridines are widely used as calcium channel blockers to treat cardiovascular diseases.
Industry: In the pharmaceutical industry, dihydropyridines are essential for developing new drugs with improved efficacy and safety profiles.
作用機序
ジヒドロピリジンは、動脈血管の平滑筋細胞にある電位依存性L型カルシウムチャネルに結合して阻害することにより、その効果を発揮します。これらのチャネルを阻害することで、ジヒドロピリジンは細胞へのカルシウムイオンの流入を防ぎ、筋弛緩と血管拡張をもたらします。 このメカニズムにより血管抵抗が低下し、血圧が低下するため、ジヒドロピリジンは高血圧やその他の心臓血管疾患の治療に効果的です .
類似の化合物との比較
ジヒドロピリジンは、ベラパミルやジルチアゼムなどの非ジヒドロピリジンを含む、より広いカルシウムチャネルブロッカーのクラスに属しています。 ジヒドロピリジンは主に血管平滑筋に影響を与え、血管拡張を促進するのに対し、非ジヒドロピリジンは心臓機能により大きな影響を与え、心拍数と収縮力を低下させます .
類似の化合物
ニフェジピン: 高血圧と狭心症の治療に使用されるジヒドロピリジン。
アムロジピン: 作用時間が長く、高血圧と狭心症に使用される別のジヒドロピリジン。
ベラパミル: 心臓と血管の両方に影響を与える非ジヒドロピリジンであり、高血圧、狭心症、不整脈に使用されます。
類似化合物との比較
Dihydropyridines are part of a broader class of calcium channel blockers, which also includes non-dihydropyridines such as verapamil and diltiazem. While dihydropyridines primarily affect vascular smooth muscle and promote vasodilation, non-dihydropyridines have a more significant impact on cardiac function, including reducing heart rate and contractility .
Similar Compounds
Nifedipine: A dihydropyridine used to treat hypertension and angina.
Amlodipine: Another this compound with a longer duration of action, used for hypertension and angina.
Verapamil: A non-dihydropyridine that affects both the heart and blood vessels, used for hypertension, angina, and arrhythmias.
Diltiazem: A non-dihydropyridine with similar uses to verapamil but with a different side effect profile.
特性
CAS番号 |
22694-45-7 |
---|---|
分子式 |
C5H7N |
分子量 |
81.12 g/mol |
IUPAC名 |
1,2-dihydropyridine |
InChI |
InChI=1S/C5H7N/c1-2-4-6-5-3-1/h1-4,6H,5H2 |
InChIキー |
MMWRGWQTAMNAFC-UHFFFAOYSA-N |
SMILES |
C1C=CC=CN1 |
正規SMILES |
C1C=CC=CN1 |
同義語 |
1,4-dihydropyridine dihydropyridine Lemildipine |
製品の起源 |
United States |
Retrosynthesis Analysis
AI-Powered Synthesis Planning: Our tool employs the Template_relevance Pistachio, Template_relevance Bkms_metabolic, Template_relevance Pistachio_ringbreaker, Template_relevance Reaxys, Template_relevance Reaxys_biocatalysis model, leveraging a vast database of chemical reactions to predict feasible synthetic routes.
One-Step Synthesis Focus: Specifically designed for one-step synthesis, it provides concise and direct routes for your target compounds, streamlining the synthesis process.
Accurate Predictions: Utilizing the extensive PISTACHIO, BKMS_METABOLIC, PISTACHIO_RINGBREAKER, REAXYS, REAXYS_BIOCATALYSIS database, our tool offers high-accuracy predictions, reflecting the latest in chemical research and data.
Strategy Settings
Precursor scoring | Relevance Heuristic |
---|---|
Min. plausibility | 0.01 |
Model | Template_relevance |
Template Set | Pistachio/Bkms_metabolic/Pistachio_ringbreaker/Reaxys/Reaxys_biocatalysis |
Top-N result to add to graph | 6 |
Feasible Synthetic Routes
試験管内研究製品の免責事項と情報
BenchChemで提示されるすべての記事および製品情報は、情報提供を目的としています。BenchChemで購入可能な製品は、生体外研究のために特別に設計されています。生体外研究は、ラテン語の "in glass" に由来し、生物体の外で行われる実験を指します。これらの製品は医薬品または薬として分類されておらず、FDAから任何の医療状態、病気、または疾患の予防、治療、または治癒のために承認されていません。これらの製品を人間または動物に体内に導入する形態は、法律により厳格に禁止されています。これらのガイドラインに従うことは、研究と実験において法的および倫理的な基準の遵守を確実にするために重要です。