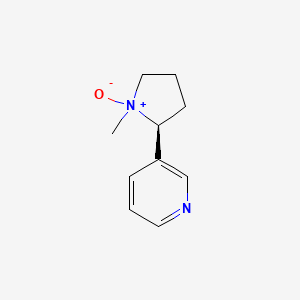
nicotine-1'-N-oxide
概要
説明
Nicotine N (1’)-oxide is a tertiary amine oxide resulting from the oxidation of the pyrrolidine nitrogen of nicotine. It is a member of pyrrolidine N-oxides and a member of pyridines .
Synthesis Analysis
The synthesis of nicotine-1’-N-oxide has been studied in various experimental systems. For instance, it has been prepared by the oxidation of (−)-nicotine with hydrogen peroxide . The analysis of nicotine-1’-N-oxide in urine, in the presence of nicotine and cotinine, has also been applied to the study of in vivo nicotine metabolism in man .Molecular Structure Analysis
The molecular structure of nicotine-1’-N-oxide has been studied using experimental IR and Raman spectra and quantum chemical studies . It has been found that nicotine-1’-N-oxide is a member of the class of pyridine N-oxides obtained by oxidation of the pyridine nitrogen of (S)-nicotine .Chemical Reactions Analysis
Nicotine-1’-N-oxide is thermally unstable and its analysis is susceptible to bias due to flavoring or coloring . It has been analyzed for the seven nicotine degradants using Enthalpy’s in-house validated LC-MS/MS method .Physical And Chemical Properties Analysis
Nicotine-1’-N-oxide has a molecular weight of 178.23 g/mol . Its IUPAC name is 3- (1-methyl-1-oxidopyrrolidin-1-ium-2-yl)pyridine .科学的研究の応用
Microbial Degradation
Nicotine-1’-N-oxide: can be degraded by specific bacterial strains, which has implications for bioremediation and the treatment of nicotine-contaminated environments. A study identified two strains of Arthrobacter globiformis that showed marked substrate specificity for nicotine-1’-N-oxide . This discovery is significant for developing microbial methods to degrade nicotine residues in agricultural settings, particularly in soil and water contaminated by tobacco industry waste.
Enzymatic Oxidation
The compound is a substrate for flavin monooxygenase enzymes (FMOs) , which play a role in nicotine metabolism . Understanding the oxidation of nicotine-1’-N-oxide by FMOs can provide insights into individual variations in nicotine addiction and clearance from the body. This knowledge is crucial for personalized medicine approaches in smoking cessation therapies.
Tobacco Smoke Condensate Analysis
The compound has been used in screening tobacco smoke condensates for nicotinic acetylcholine receptor ligands . This application is important for identifying harmful compounds in cigarette smoke and could lead to the development of safer tobacco products or smoking cessation aids.
作用機序
- In the brain, nicotine-1’-N-oxide binds to nAChRs on dopaminergic neurons within the cortico-limbic pathways .
- Activation of nAChRs leads to downstream signaling events, including changes in intracellular calcium levels and neurotransmitter release .
- Nicotine-1’-N-oxide affects several pathways:
Target of Action
Mode of Action
Biochemical Pathways
Pharmacokinetics
Result of Action
Action Environment
Safety and Hazards
Nicotine-1’-N-oxide should be handled with care. Avoid breathing mist, gas or vapors. Avoid contact with skin and eyes. Use personal protective equipment. Wear chemical impermeable gloves. Ensure adequate ventilation. Remove all sources of ignition. Evacuate personnel to safe areas. Keep people away from and upwind of spill/leak .
特性
IUPAC Name |
3-[(2S)-1-methyl-1-oxidopyrrolidin-1-ium-2-yl]pyridine | |
---|---|---|
Source | PubChem | |
URL | https://pubchem.ncbi.nlm.nih.gov | |
Description | Data deposited in or computed by PubChem | |
InChI |
InChI=1S/C10H14N2O/c1-12(13)7-3-5-10(12)9-4-2-6-11-8-9/h2,4,6,8,10H,3,5,7H2,1H3/t10-,12?/m0/s1 | |
Source | PubChem | |
URL | https://pubchem.ncbi.nlm.nih.gov | |
Description | Data deposited in or computed by PubChem | |
InChI Key |
RWFBQHICRCUQJJ-NUHJPDEHSA-N | |
Source | PubChem | |
URL | https://pubchem.ncbi.nlm.nih.gov | |
Description | Data deposited in or computed by PubChem | |
Canonical SMILES |
C[N+]1(CCCC1C2=CN=CC=C2)[O-] | |
Source | PubChem | |
URL | https://pubchem.ncbi.nlm.nih.gov | |
Description | Data deposited in or computed by PubChem | |
Isomeric SMILES |
C[N+]1(CCC[C@H]1C2=CN=CC=C2)[O-] | |
Source | PubChem | |
URL | https://pubchem.ncbi.nlm.nih.gov | |
Description | Data deposited in or computed by PubChem | |
Molecular Formula |
C10H14N2O | |
Source | PubChem | |
URL | https://pubchem.ncbi.nlm.nih.gov | |
Description | Data deposited in or computed by PubChem | |
DSSTOX Substance ID |
DTXSID70867021 | |
Record name | (-)-Nicotine 1′-N-oxide | |
Source | EPA DSSTox | |
URL | https://comptox.epa.gov/dashboard/DTXSID70867021 | |
Description | DSSTox provides a high quality public chemistry resource for supporting improved predictive toxicology. | |
Molecular Weight |
178.23 g/mol | |
Source | PubChem | |
URL | https://pubchem.ncbi.nlm.nih.gov | |
Description | Data deposited in or computed by PubChem | |
Product Name |
nicotine-1'-N-oxide | |
CAS RN |
491-26-9 | |
Record name | Nicotine 1′-N-oxide | |
Source | CAS Common Chemistry | |
URL | https://commonchemistry.cas.org/detail?cas_rn=491-26-9 | |
Description | CAS Common Chemistry is an open community resource for accessing chemical information. Nearly 500,000 chemical substances from CAS REGISTRY cover areas of community interest, including common and frequently regulated chemicals, and those relevant to high school and undergraduate chemistry classes. This chemical information, curated by our expert scientists, is provided in alignment with our mission as a division of the American Chemical Society. | |
Explanation | The data from CAS Common Chemistry is provided under a CC-BY-NC 4.0 license, unless otherwise stated. | |
Record name | Nicotine N'-oxide | |
Source | ChemIDplus | |
URL | https://pubchem.ncbi.nlm.nih.gov/substance/?source=chemidplus&sourceid=0000491269 | |
Description | ChemIDplus is a free, web search system that provides access to the structure and nomenclature authority files used for the identification of chemical substances cited in National Library of Medicine (NLM) databases, including the TOXNET system. | |
Record name | (-)-Nicotine 1′-N-oxide | |
Source | EPA DSSTox | |
URL | https://comptox.epa.gov/dashboard/DTXSID70867021 | |
Description | DSSTox provides a high quality public chemistry resource for supporting improved predictive toxicology. | |
Record name | NICOTINE N'-OXIDE | |
Source | FDA Global Substance Registration System (GSRS) | |
URL | https://gsrs.ncats.nih.gov/ginas/app/beta/substances/9R173T257I | |
Description | The FDA Global Substance Registration System (GSRS) enables the efficient and accurate exchange of information on what substances are in regulated products. Instead of relying on names, which vary across regulatory domains, countries, and regions, the GSRS knowledge base makes it possible for substances to be defined by standardized, scientific descriptions. | |
Explanation | Unless otherwise noted, the contents of the FDA website (www.fda.gov), both text and graphics, are not copyrighted. They are in the public domain and may be republished, reprinted and otherwise used freely by anyone without the need to obtain permission from FDA. Credit to the U.S. Food and Drug Administration as the source is appreciated but not required. | |
Synthesis routes and methods
Procedure details
Retrosynthesis Analysis
AI-Powered Synthesis Planning: Our tool employs the Template_relevance Pistachio, Template_relevance Bkms_metabolic, Template_relevance Pistachio_ringbreaker, Template_relevance Reaxys, Template_relevance Reaxys_biocatalysis model, leveraging a vast database of chemical reactions to predict feasible synthetic routes.
One-Step Synthesis Focus: Specifically designed for one-step synthesis, it provides concise and direct routes for your target compounds, streamlining the synthesis process.
Accurate Predictions: Utilizing the extensive PISTACHIO, BKMS_METABOLIC, PISTACHIO_RINGBREAKER, REAXYS, REAXYS_BIOCATALYSIS database, our tool offers high-accuracy predictions, reflecting the latest in chemical research and data.
Strategy Settings
Precursor scoring | Relevance Heuristic |
---|---|
Min. plausibility | 0.01 |
Model | Template_relevance |
Template Set | Pistachio/Bkms_metabolic/Pistachio_ringbreaker/Reaxys/Reaxys_biocatalysis |
Top-N result to add to graph | 6 |
Feasible Synthetic Routes
試験管内研究製品の免責事項と情報
BenchChemで提示されるすべての記事および製品情報は、情報提供を目的としています。BenchChemで購入可能な製品は、生体外研究のために特別に設計されています。生体外研究は、ラテン語の "in glass" に由来し、生物体の外で行われる実験を指します。これらの製品は医薬品または薬として分類されておらず、FDAから任何の医療状態、病気、または疾患の予防、治療、または治癒のために承認されていません。これらの製品を人間または動物に体内に導入する形態は、法律により厳格に禁止されています。これらのガイドラインに従うことは、研究と実験において法的および倫理的な基準の遵守を確実にするために重要です。