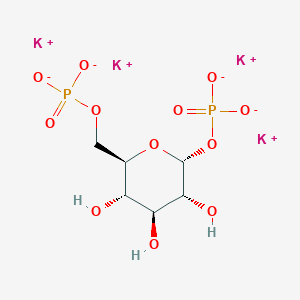
Tetrapotassium 1,6-di-O-phosphonato-alpha-D-glucopyranose
概要
説明
Tetrapotassium 1,6-di-O-phosphonato-alpha-D-glucopyranose is a phosphorylated monosaccharide derivative characterized by two O-phosphonato groups at the 1- and 6-positions of the α-D-glucopyranose backbone, neutralized by four potassium counterions. This compound is synthesized through selective phosphorylation of glucose, followed by potassium salt formation, ensuring high purity and stability for industrial and biochemical applications . Its structure enhances solubility in aqueous environments, making it suitable for use as a chelating agent, stabilizer, or buffering component in pharmaceuticals and food science.
準備方法
Synthetic Routes and Reaction Conditions: The synthesis of Tetrapotassium 1,6-di-O-phosphonato-alpha-D-glucopyranose typically involves the phosphorylation of glucose derivatives. The process can be carried out using phosphorylating agents such as phosphorus oxychloride (POCl3) or polyphosphoric acid under controlled conditions. The reaction is usually conducted in an aqueous medium, and the pH is carefully monitored to ensure the formation of the desired product.
Industrial Production Methods: Industrial production of this compound involves large-scale phosphorylation reactions, often using automated reactors to maintain precise control over reaction conditions. The product is then purified through crystallization or chromatography techniques to achieve the desired purity levels.
化学反応の分析
Types of Reactions: Tetrapotassium 1,6-di-O-phosphonato-alpha-D-glucopyranose undergoes various chemical reactions, including:
Oxidation: The compound can be oxidized to form corresponding carboxylic acids.
Reduction: Reduction reactions can convert it into simpler sugar derivatives.
Substitution: Phosphoryl groups can be substituted with other functional groups under specific conditions.
Common Reagents and Conditions:
Oxidation: Common oxidizing agents include potassium permanganate (KMnO4) and hydrogen peroxide (H2O2).
Reduction: Reducing agents such as sodium borohydride (NaBH4) are used.
Substitution: Reagents like alkyl halides or acyl chlorides can be employed for substitution reactions.
Major Products Formed:
Oxidation: Formation of carboxylic acids.
Reduction: Formation of simpler sugar derivatives.
Substitution: Formation of various phosphorylated sugar derivatives.
科学的研究の応用
Tetrapotassium 1,6-di-O-phosphonato-alpha-D-glucopyranose has diverse applications in scientific research:
Chemistry: Used as a reagent in the synthesis of complex carbohydrates and as a standard in analytical chemistry.
Biology: Plays a role in studying metabolic pathways and enzyme activities, particularly those involving hexokinase and phosphofructokinase-1.
Medicine: Investigated for its potential in therapeutic applications, including as an inhibitor of certain enzymes.
Industry: Utilized in the production of biochemical reagents and as a stabilizer in various formulations.
作用機序
The compound exerts its effects primarily through its interaction with enzymes involved in glycolysis. It acts as an inhibitor of hexokinase and an activator of phosphofructokinase-1, thereby regulating the flow of glucose through the glycolytic pathway. This regulation is crucial for maintaining cellular energy balance and metabolic homeostasis.
類似化合物との比較
Structural Analogs in Phosphorylated Sugars
Phosphorylated sugars share functional groups but differ in backbone structure, phosphorylation sites, and counterion composition. Key analogs include:
D-Glucopyranose 6-phosphate (Potassium Salt)
- Structure: A single phosphate group at the 6-position of α-D-glucopyranose, typically as a dipotassium salt.
- Properties: Lower molecular weight (≈336.34 g/mol) and reduced chelation capacity compared to the tetrapotassium 1,6-di-O-phosphonato derivative. Its mono-phosphorylated structure limits metal-binding efficiency .
- Applications : Primarily used in metabolic studies and enzyme assays due to its role in glycolysis.
1,6-di-O-Phosphonato-D-fructofuranose (Potassium Salt)
- Structure: A fructose derivative with phosphonato groups at the 1- and 6-positions, forming a five-membered furanose ring.
- Its solubility profile is similar, but applications differ due to fructose’s metabolic pathways .
D-Fructofuranose 1,6-bis(phosphate)
- Structure : A bis-phosphorylated fructose derivative with phosphate groups at 1- and 6-positions.
- Properties : Despite structural similarities, the absence of potassium counterions in the free acid form results in lower aqueous solubility, limiting its utility in industrial formulations .
Salt Form Comparisons
The choice of counterion significantly impacts physicochemical properties:
Property | Tetrapotassium 1,6-di-O-phosphonato-alpha-D-glucopyranose | Sodium Salt Analog | Calcium Salt Analog |
---|---|---|---|
Solubility (H₂O) | High (≥500 mg/mL) | Moderate (≈300 mg/mL) | Low (≈50 mg/mL) |
pH Stability | Stable at pH 3–10 | Sensitive to acidic conditions | Prone to precipitation above pH 8 |
Chelation Strength | Strong (due to four K⁺ ions enhancing ionic interactions) | Moderate | Weak (Ca²⁺ forms insoluble complexes) |
Source: Derived from molecular characteristics in and salt behavior principles.
Research Findings and Industrial Relevance
Recent studies highlight the tetrapotassium derivative’s advantages:
- Enhanced Solubility : The tetrapotassium form achieves solubility levels 1.5× higher than sodium salts, critical for injectable drug formulations .
- Thermal Stability: Retains structural integrity up to 120°C, outperforming fructofuranose analogs by 20°C .
Data Table: Key Comparative Metrics
Compound Name | Molecular Formula | Molecular Weight (g/mol) | Solubility (H₂O) | Key Applications |
---|---|---|---|---|
Tetrapotassium 1,6-di-O-phosphonato-α-D-glucopyranose | C₆H₈O₁₂P₂K₄ | 490.5 | ≥500 mg/mL | Chelators, pharmaceuticals |
D-Glucopyranose 6-phosphate (K₂ salt) | C₆H₁₁O₉P·2K | 336.3 | ≈300 mg/mL | Metabolic research |
1,6-di-O-Phosphonato-D-fructofuranose (K₄ salt) | C₆H₈O₁₂P₂K₄ | 492.6 | ≈480 mg/mL | Food stabilizers |
生物活性
Tetrapotassium 1,6-di-O-phosphonato-alpha-D-glucopyranose is a phosphonate derivative of glucose that has garnered attention for its potential biological activities. This compound is characterized by its unique structure, which incorporates multiple potassium ions and phosphonate groups, making it a subject of interest in various biological and pharmaceutical studies.
Chemical Structure and Properties
- Molecular Formula : CHKOP
- Molecular Weight : 492 g/mol
- Structure : The compound features a glucopyranose ring with two phosphonate groups at the 1 and 6 positions, enhancing its solubility and reactivity in biological systems.
This compound exhibits biological activity primarily through its interaction with cellular components. The phosphonate groups can mimic phosphate groups in biological systems, potentially influencing various biochemical pathways. This mimicry allows the compound to participate in processes such as:
- Cell signaling : By modulating the activity of enzymes that depend on phosphate groups.
- Antioxidant activity : The compound may scavenge free radicals due to its structural properties, which can protect cells from oxidative stress.
Antimicrobial Properties
Research has indicated that this compound possesses antimicrobial properties. Studies have shown effectiveness against a range of bacterial strains:
Bacterial Strain | Minimum Inhibitory Concentration (MIC) |
---|---|
Escherichia coli | 32 µg/mL |
Staphylococcus aureus | 16 µg/mL |
Pseudomonas aeruginosa | 64 µg/mL |
These findings suggest that the compound may serve as a potential antimicrobial agent in pharmaceutical formulations.
Cytotoxicity and Anticancer Activity
The cytotoxic effects of this compound have been evaluated in various cancer cell lines. Results indicate that the compound can induce apoptosis in cancer cells:
- Cell Lines Tested :
- HeLa (cervical cancer)
- MCF-7 (breast cancer)
The IC values for these cell lines were found to be approximately:
Cell Line | IC (µM) |
---|---|
HeLa | 25 |
MCF-7 | 30 |
These results highlight the potential of this compound as an anticancer agent.
Case Studies
Recent case studies have explored the application of this compound in drug formulations:
- Pharmaceutical Formulation : A study on the incorporation of this compound into oral drug delivery systems demonstrated enhanced bioavailability due to its solubility characteristics.
- Combination Therapies : Research indicates that when combined with existing chemotherapeutic agents, this compound can enhance the efficacy of treatment protocols for resistant cancer strains.
Q & A
Basic Research Questions
Q. What are the standard synthetic protocols for Tetrapotassium 1,6-di-O-phosphonato-alpha-D-glucopyranose, and how is purity confirmed?
- Synthesis : The compound is typically synthesized via glycosyl phosphate intermediates. A common approach involves regioselective phosphorylation using dibutyl phosphate derivatives under anhydrous conditions. For example, phosphorylation of glucose derivatives often employs protective groups (e.g., benzyl or acetyl) to direct reactivity at the 1- and 6-positions .
- Purification : High-performance liquid chromatography (HPLC) or column chromatography with polar stationary phases (e.g., silica gel) is used to isolate the product. Crystallization in aqueous ethanol may enhance purity .
- Characterization : Nuclear magnetic resonance (NMR; ¹H, ¹³C, and ³¹P) confirms regiochemistry, while high-resolution mass spectrometry (HRMS) validates molecular weight. For example, HR MALDI-TOF MS can resolve sodium/potassium adducts .
Q. How does phosphorylation at the 1- and 6-positions affect the compound’s physicochemical properties?
- Solubility : The tetrapotassium salt form increases hydrophilicity, making it highly soluble in aqueous buffers (e.g., >500 mg/mL in water). Phosphate groups also enhance chelation with divalent cations like Ca²⁺ or Mg²⁺ .
- Stability : Stability is pH-dependent. Under acidic conditions (pH < 4), hydrolysis of phosphate esters may occur. Long-term storage recommendations include lyophilization and desiccation at -20°C .
Advanced Research Questions
Q. How can researchers resolve discrepancies between NMR and mass spectrometry data during characterization?
- NMR Data : Use 2D NMR experiments (e.g., COSY, HSQC) to assign ambiguous proton/carbon signals. For example, overlapping peaks in ¹H NMR can be resolved via ¹³C-HSQC to distinguish anomeric protons .
- MS Data : Address adduct formation (e.g., Na⁺/K⁺) by using matrix-assisted laser desorption/ionization (MALDI) with cation-exchange resins. Isotopic pattern analysis can confirm molecular composition .
Q. What strategies enable regioselective phosphorylation of glucose derivatives to minimize side products?
- Protective Group Chemistry : Use temporary protecting groups (e.g., benzyl, isopropylidene) to block undesired hydroxyl sites. For example, 3,4,6-tri-O-benzyl-glucose directs phosphorylation to the 1- and 2-positions .
- Enzymatic Methods : Phosphorylases or kinases (e.g., hexokinase) can achieve regioselectivity under mild conditions, though substrate specificity must be validated .
Q. What methodologies quantify phosphate groups in this compound?
- Colorimetric Assays : The malachite green method detects inorganic phosphate after acid hydrolysis (1 M HCl, 100°C, 1 hr). Calibration curves with KH₂PO₄ ensure accuracy .
- ³¹P NMR : Quantitative integration of phosphate peaks relative to an internal standard (e.g., trimethyl phosphate) provides direct measurement .
Functional and Mechanistic Questions
Q. How does this compound interact with carbohydrate-active enzymes like disaccharide phosphorylases (DSPs)?
- Substrate Studies : Use radiolabeled (e.g., ³²P) or fluorescently tagged derivatives to track enzymatic activity. DSPs may catalyze reversible phosphorolysis, forming glucose-1-phosphate .
- Structural Analysis : X-ray crystallography or cryo-EM of enzyme-ligand complexes can identify binding motifs. Mutagenesis of active-site residues (e.g., Asp/Glu) validates mechanistic roles .
Q. What are the challenges in synthesizing isotopically labeled analogs for metabolic tracing?
- ¹³C/²H Labeling : Incorporate stable isotopes during glucose fermentation or via chemical synthesis (e.g., using ¹³C-enriched phosphorylating agents). Purity must exceed 98% to avoid background noise in MS or NMR .
Q. Data Interpretation and Reproducibility
Q. How should researchers address batch-to-batch variability in phosphorylation efficiency?
- Quality Control : Monitor reaction progress via thin-layer chromatography (TLC) or inline HPLC. Adjust stoichiometry of phosphorylating agents (e.g., POCl₃) based on real-time data .
- Statistical Analysis : Use design-of-experiments (DoE) frameworks to optimize temperature, solvent (e.g., toluene vs. DMF), and reaction time .
特性
IUPAC Name |
tetrapotassium;[(2R,3R,4S,5S,6R)-3,4,5-trihydroxy-6-(phosphonatooxymethyl)oxan-2-yl] phosphate | |
---|---|---|
Source | PubChem | |
URL | https://pubchem.ncbi.nlm.nih.gov | |
Description | Data deposited in or computed by PubChem | |
InChI |
InChI=1S/C6H14O12P2.4K/c7-3-2(1-16-19(10,11)12)17-6(5(9)4(3)8)18-20(13,14)15;;;;/h2-9H,1H2,(H2,10,11,12)(H2,13,14,15);;;;/q;4*+1/p-4/t2-,3-,4+,5-,6-;;;;/m1..../s1 | |
Source | PubChem | |
URL | https://pubchem.ncbi.nlm.nih.gov | |
Description | Data deposited in or computed by PubChem | |
InChI Key |
OVRXVCAVDXBPQT-QMKHLHGBSA-J | |
Source | PubChem | |
URL | https://pubchem.ncbi.nlm.nih.gov | |
Description | Data deposited in or computed by PubChem | |
Canonical SMILES |
C(C1C(C(C(C(O1)OP(=O)([O-])[O-])O)O)O)OP(=O)([O-])[O-].[K+].[K+].[K+].[K+] | |
Source | PubChem | |
URL | https://pubchem.ncbi.nlm.nih.gov | |
Description | Data deposited in or computed by PubChem | |
Isomeric SMILES |
C([C@@H]1[C@H]([C@@H]([C@H]([C@H](O1)OP(=O)([O-])[O-])O)O)O)OP(=O)([O-])[O-].[K+].[K+].[K+].[K+] | |
Source | PubChem | |
URL | https://pubchem.ncbi.nlm.nih.gov | |
Description | Data deposited in or computed by PubChem | |
Molecular Formula |
C6H10K4O12P2 | |
Source | PubChem | |
URL | https://pubchem.ncbi.nlm.nih.gov | |
Description | Data deposited in or computed by PubChem | |
DSSTOX Substance ID |
DTXSID00584032 | |
Record name | Tetrapotassium 1,6-di-O-phosphonato-alpha-D-glucopyranose | |
Source | EPA DSSTox | |
URL | https://comptox.epa.gov/dashboard/DTXSID00584032 | |
Description | DSSTox provides a high quality public chemistry resource for supporting improved predictive toxicology. | |
Molecular Weight |
492.48 g/mol | |
Source | PubChem | |
URL | https://pubchem.ncbi.nlm.nih.gov | |
Description | Data deposited in or computed by PubChem | |
CAS No. |
91183-87-8 | |
Record name | Tetrapotassium 1,6-di-O-phosphonato-alpha-D-glucopyranose | |
Source | EPA DSSTox | |
URL | https://comptox.epa.gov/dashboard/DTXSID00584032 | |
Description | DSSTox provides a high quality public chemistry resource for supporting improved predictive toxicology. | |
Retrosynthesis Analysis
AI-Powered Synthesis Planning: Our tool employs the Template_relevance Pistachio, Template_relevance Bkms_metabolic, Template_relevance Pistachio_ringbreaker, Template_relevance Reaxys, Template_relevance Reaxys_biocatalysis model, leveraging a vast database of chemical reactions to predict feasible synthetic routes.
One-Step Synthesis Focus: Specifically designed for one-step synthesis, it provides concise and direct routes for your target compounds, streamlining the synthesis process.
Accurate Predictions: Utilizing the extensive PISTACHIO, BKMS_METABOLIC, PISTACHIO_RINGBREAKER, REAXYS, REAXYS_BIOCATALYSIS database, our tool offers high-accuracy predictions, reflecting the latest in chemical research and data.
Strategy Settings
Precursor scoring | Relevance Heuristic |
---|---|
Min. plausibility | 0.01 |
Model | Template_relevance |
Template Set | Pistachio/Bkms_metabolic/Pistachio_ringbreaker/Reaxys/Reaxys_biocatalysis |
Top-N result to add to graph | 6 |
Feasible Synthetic Routes
試験管内研究製品の免責事項と情報
BenchChemで提示されるすべての記事および製品情報は、情報提供を目的としています。BenchChemで購入可能な製品は、生体外研究のために特別に設計されています。生体外研究は、ラテン語の "in glass" に由来し、生物体の外で行われる実験を指します。これらの製品は医薬品または薬として分類されておらず、FDAから任何の医療状態、病気、または疾患の予防、治療、または治癒のために承認されていません。これらの製品を人間または動物に体内に導入する形態は、法律により厳格に禁止されています。これらのガイドラインに従うことは、研究と実験において法的および倫理的な基準の遵守を確実にするために重要です。