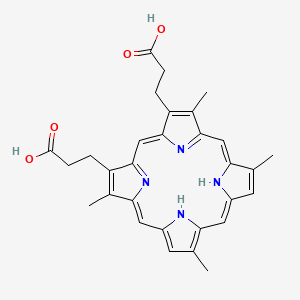
Deuteroporphyrin
- 専門家チームからの見積もりを受け取るには、QUICK INQUIRYをクリックしてください。
- 品質商品を競争力のある価格で提供し、研究に集中できます。
説明
Deuteroporphyrin IX is a member of porphyrins.
This compound is a natural product found in Corallistes with data available.
科学的研究の応用
Synthesis and Properties
- Deuteroporphyrin and its complexes, such as those with iron(III), have been synthesized and characterized, providing insights into their thermal properties and phase transitions (Berezin et al., 2013).
Biomimetic Catalysis
- Metallo-deuteroporphyrins, modeled after the cytochrome P450 enzyme, have been used as catalysts for the oxidation of lignin to aromatics, demonstrating significant catalytic activity and stability (Zhu et al., 2015).
Photodynamic Research
- This compound IX derivatives have shown significant photodynamic effects on single neurons, demonstrating potential for applications in neuroscience and related fields (Uzdensky et al., 2001).
Synthesis Methods
- Efficient methods for synthesizing this compound derivatives have been developed, providing new pathways for producing these compounds in various forms (Hu et al., 2010).
Electrochemical Applications
- Co(II) this compound has been utilized in electrochemical sensors, especially for the potentiometric determination of nitrite, indicating its utility in analytical chemistry (Cosnier et al., 2003).
Protein Interactions
- The interaction of this compound with proteins like albumin has been studied, revealing insights into molecular mechanisms of aggregation and binding affinities (Lebedeva et al., 2020).
Oxidation Catalysis
- Metallo-deuteroporphyrins have been found effective in catalyzing the oxidation of organic compounds like cyclohexane, offering potential in industrial applications (Hu et al., 2008).
特性
CAS番号 |
448-65-7 |
---|---|
分子式 |
C30H30N4O4 |
分子量 |
510.6 g/mol |
IUPAC名 |
3-[18-(2-carboxyethyl)-3,8,13,17-tetramethyl-22,23-dihydroporphyrin-2-yl]propanoic acid |
InChI |
InChI=1S/C30H30N4O4/c1-15-9-20-12-25-17(3)21(5-7-29(35)36)27(33-25)14-28-22(6-8-30(37)38)18(4)26(34-28)13-24-16(2)10-19(32-24)11-23(15)31-20/h9-14,31-32H,5-8H2,1-4H3,(H,35,36)(H,37,38) |
InChIキー |
VAJVGAQAYOAJQI-UHFFFAOYSA-N |
SMILES |
CC1=CC2=CC3=C(C=C(N3)C=C4C(=C(C(=N4)C=C5C(=C(C(=N5)C=C1N2)C)CCC(=O)O)CCC(=O)O)C)C |
正規SMILES |
CC1=CC2=CC3=C(C=C(N3)C=C4C(=C(C(=N4)C=C5C(=C(C(=N5)C=C1N2)C)CCC(=O)O)CCC(=O)O)C)C |
関連するCAS |
68929-05-5 (di-hydrochloride) |
同義語 |
deuteroporphyrin deuteroporphyrin-IX deuteroporphyrin-IX dihydrochloride |
製品の起源 |
United States |
Retrosynthesis Analysis
AI-Powered Synthesis Planning: Our tool employs the Template_relevance Pistachio, Template_relevance Bkms_metabolic, Template_relevance Pistachio_ringbreaker, Template_relevance Reaxys, Template_relevance Reaxys_biocatalysis model, leveraging a vast database of chemical reactions to predict feasible synthetic routes.
One-Step Synthesis Focus: Specifically designed for one-step synthesis, it provides concise and direct routes for your target compounds, streamlining the synthesis process.
Accurate Predictions: Utilizing the extensive PISTACHIO, BKMS_METABOLIC, PISTACHIO_RINGBREAKER, REAXYS, REAXYS_BIOCATALYSIS database, our tool offers high-accuracy predictions, reflecting the latest in chemical research and data.
Strategy Settings
Precursor scoring | Relevance Heuristic |
---|---|
Min. plausibility | 0.01 |
Model | Template_relevance |
Template Set | Pistachio/Bkms_metabolic/Pistachio_ringbreaker/Reaxys/Reaxys_biocatalysis |
Top-N result to add to graph | 6 |
Feasible Synthetic Routes
試験管内研究製品の免責事項と情報
BenchChemで提示されるすべての記事および製品情報は、情報提供を目的としています。BenchChemで購入可能な製品は、生体外研究のために特別に設計されています。生体外研究は、ラテン語の "in glass" に由来し、生物体の外で行われる実験を指します。これらの製品は医薬品または薬として分類されておらず、FDAから任何の医療状態、病気、または疾患の予防、治療、または治癒のために承認されていません。これらの製品を人間または動物に体内に導入する形態は、法律により厳格に禁止されています。これらのガイドラインに従うことは、研究と実験において法的および倫理的な基準の遵守を確実にするために重要です。